Abstract
Aims
Animal models have been developed that allow simulation of post-traumatic joint contracture. One such model involves contracture-forming surgery followed by surgical capsular release. This model allows testing of antifibrotic agents, such as rosiglitazone.
Methods
A total of 20 rabbits underwent contracture-forming surgery. Eight weeks later, the animals underwent a surgical capsular release. Ten animals received rosiglitazone (intramuscular initially, then orally). The animals were sacrificed following 16 weeks of free cage mobilisation. The joints were tested biomechanically, and the posterior capsule was assessed histologically and via genetic microarray analysis.
Results
There was no significant difference in post-traumatic contracture between the rosiglitazone and control groups (33° (standard deviation (sd) 11) vs 37° (sd14), respectively; p = 0.4). There was no difference in number or percentage of myofibroblasts. Importantly, there were ten genes and 17 pathways that were significantly modulated by rosiglitazone in the posterior capsule.
Discussion
Rosiglitazone significantly altered the genetic expression of the posterior capsular tissue in a rabbit model, with ten genes and 17 pathways demonstrating significant modulation. However, there was no significant effect on biomechanical or histological properties.
Cite this article: M. P. Abdel. Effectiveness of rosiglitazone in reducing flexion contracture in a rabbit model of arthrofibrosis with surgical capsular release: A biomechanical, histological, and genetic analysis. Bone Joint Res 2016;5:11–17. doi: 10.1302/2046-3758.51.2000593
Article focus
-
Posttraumatic joint fibrosis is a common cause of disability and complication after joint surgery.
-
Peroxisome proliferator-activated receptor-gamma (PPAR-γ) agonists have shown antifibrotic effects in other organ systems.
-
(PPAR-γ) agonists may reduce posttraumatic fibrosis in an animal model of fibrosis.
Key messages
-
There was no significant reduction in biomechanical or histological fibrosis in animals treated with rosiglitazone in this model.
-
Potentially antifibrotic genetic changes were observed after treatment with rosiglitazone in this animal model.
Strengths and limitations
-
This study examined histological, biomechanical, and genetic responses to rosiglitazone in a standardised animal model.
-
Adequate drug dosing and delivery are not quantitated in this translational fibrosis model.
Introduction
Fibrosis can be a devastating complication secondary to trauma or surgical insult. The knee and elbow joints are particularly susceptible to post-traumatic contractures.1,2 An animal model has been recently developed and used to simulate joint contractures.3-7 This model mimics the biomechanical and histological presentation of clinical contracture.5,7-11
Clinically, patients who have post-traumatic elbow contractures typically undergo a period of rehabilitation in order to attempt to improve range of movement (ROM).1,8-11 If this fails, surgical procedures (open or arthroscopic capsular excision) are undertaken.9-13 This typically results in excellent recovery of movement in the operating room, with improved post-operative ROM. Final ROM often falls short of that gained in the operating room.10,11 Recently, our group has modified the previously used model to include a contracture release.14 In this model, joint contracture is nearly eliminated at the time of surgical contracture release, but significant recurrent contracture occurs at final time points. This model may more closely mimic the clinical situation, which involves the formation of fibrosis in spite of joint remobilisation.
Pharmacologic inhibitors of arthrofibrosis may reduce the formation of fibrosis in vivo. Transforming growth factor – beta (TGF-β) has been strongly implicated in the pathogenesis of fibrosis, both in joint contracture and other disease states.3,4,15,16 Efforts to modulate TGF-β have been effective in decreasing fibrosis in these diseases. One class of agents that has been used clinically as inhibitors of TGF-β is peroxisome proliferator-activated receptor - gamma (PPAR-γ) agonists. Several in vitro studies have demonstrated the ability of PPAR-γ agonists to decrease fibrosis.17-20In vivo effectiveness has also been clearly demonstrated in a scleroderma model, using rosiglitazone, a common oral medication for the treatment of diabetes.19,21
Pharmacological agents with antifibrogenic activity would be an invaluable tool in the treatment of arthrofibrosis. The effectiveness of these agents may be augmented by delivering them in therapeutic windows, such as at the time of a surgical capsular release. As such, we sought to determine the effectiveness of rosiglitazone in reducing joint contracture by assessing: the biomechanical effects of rosiglitazone by measuring the immediate post-operative and final joint contractures following 16 weeks of treatment; the histological effects of rosiglitazone by measuring the myofibroblast count in the capsular tissue; and the genetic effects of rosiglitazone by using a novel rabbit gene microarray.
Materials and Methods
Following institutional approval, 20 skeletally mature New Zealand White female rabbits were randomly divided into two study groups (control and rosiglitazone). Rabbits in each group underwent the same primary operation, which involves a posterior capsular injury, and pinning of the joint in full flexion, as described in previous studies (Fig. 1).7 The animals were allowed free cage activity for eight weeks following this operation.
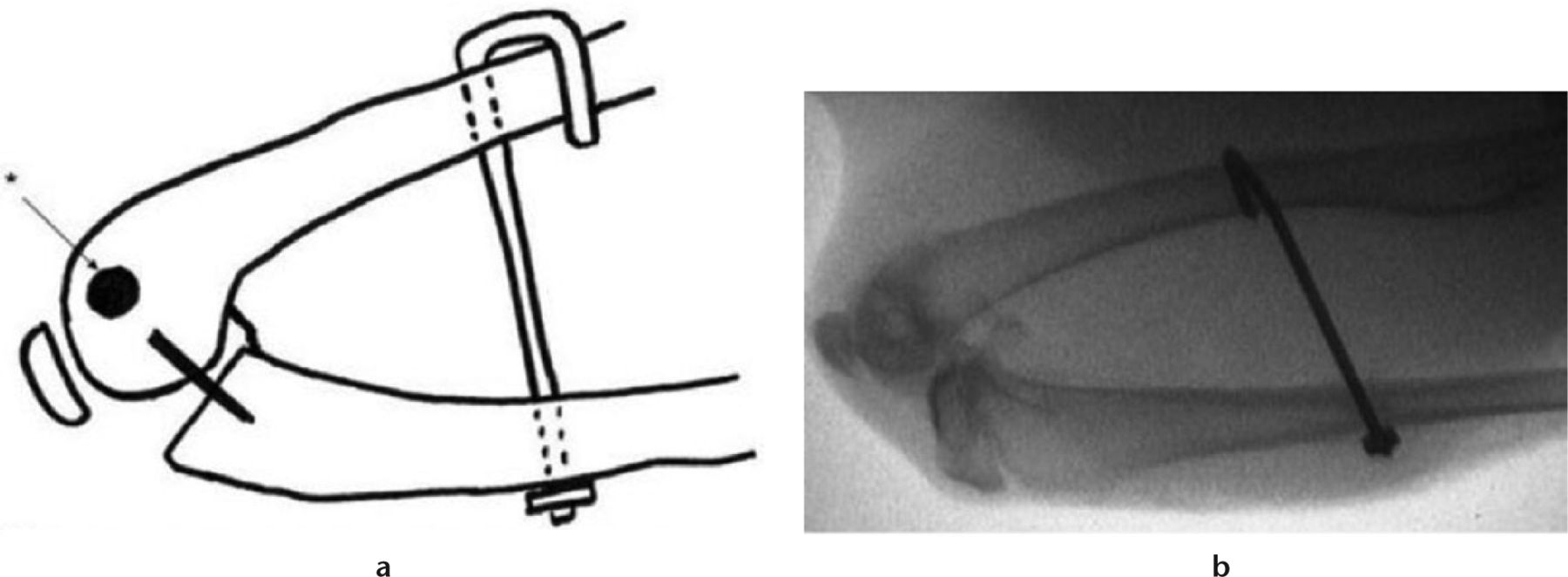
Fig. 1
Intra-operative fluoroscopy of operative limb before Kirschner wire removal.
Animals in both groups underwent a second operation eight weeks after the primary operation. The surgeons were blinded to control versus rosiglitazone group assignment. This second operation included removal of the Kirschner wire (K-wire), and a capsular release (elevation of posterior capsule under tension) as per previously described protocols.14
Intra-operative fluoroscopic images were obtained of all animals. Images were taken of the contralateral limb in gentle forced extension, the operative limb before K-wire removal, and the operative limb following the capsular release procedure as described above, in gentle forced extension (Fig. 2). Flexion contracture angles were measured on these images. This was calculated by subtracting the operative limb from the non-operative, contralateral limb. Flexion contracture was assessed for animals before K-wire removal and after capsular release.
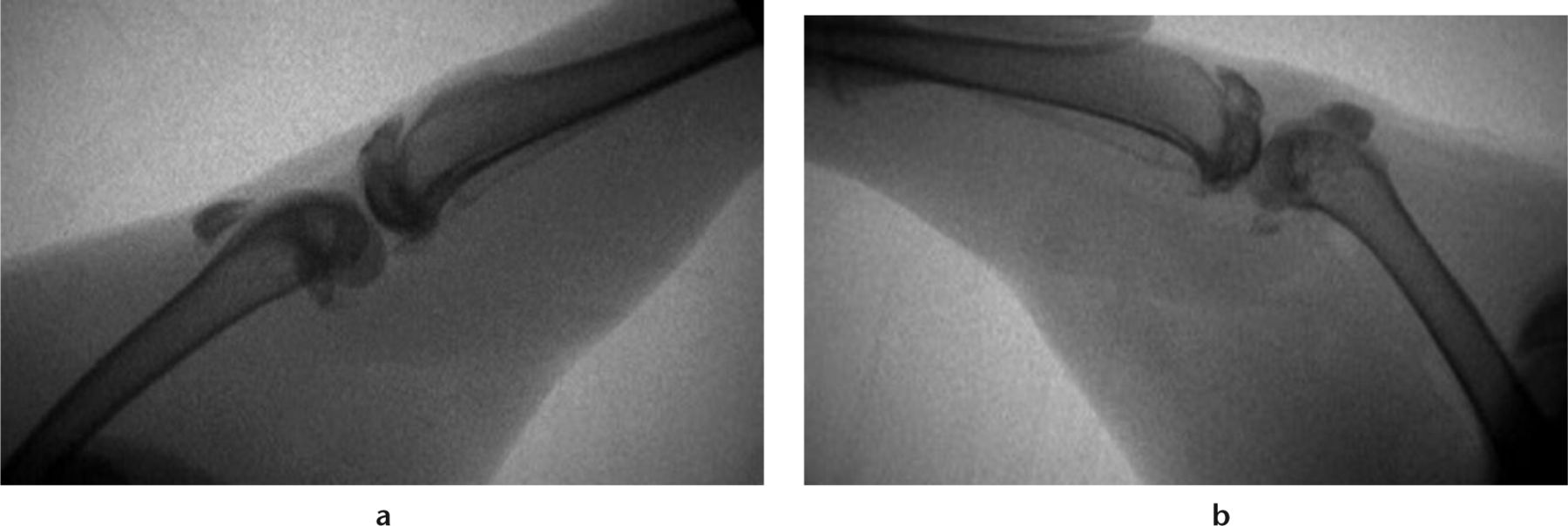
Fig. 2
Radiographs of a) the contralateral limb in gentle extension and b) the operative limb following limited capsular release, with a gentle extension force. (From Barlow JD, Hartzler RU, Abdel MP, et al. Surgical capsular release reduces flexion contracture in a rabbit model of arthrofibrosis. J Orthop Res 2013;31:1529-1532).14
Animals in the rosiglitazone group were given 3 mg/kg in dimethyl sulfoxide (DMSO) vehicle as a subcutaneous injection on the day of surgery and on post-operative day one, as oral intake is inconsistent in the peri-operative period. Animals in the control group received placebo injections on the day of surgery and on post-operative day one (DMSO vehicle alone). Beginning on post-operative day two, animals in the rosiglitazone group were given 3 mg/kg orally each day (in apple segments) until sacrifice. Animals in the control group were given placebo daily (apple segments alone) until sacrifice. Compliance with the drug regimen and placebo were high, with no animals being excluded for lack of ingestion of the drug. All animals were closely monitored by veterinary staff. There were no side effects noted from the drug or placebo. No animal had to be withdrawn from the study secondary to drug administration or reaction.
All animals were allowed free cage mobilisation (0.19 m3) for 16 weeks. Following 16 weeks of remobilisation, all animals were sacrificed under anaesthesia. The joints were immediately tested using a validated and custom-made testing device (Fig. 3). This device allows mounting of the femora and tibia to fixed points. An extension torque was then applied to the tibia through a pulley at 1° per second to a maximum torque of 20 Ncm.7 This device measures the flexion contracture of the limb, and is calibrated using standardised weights. It has been documented in the literature by our group.7
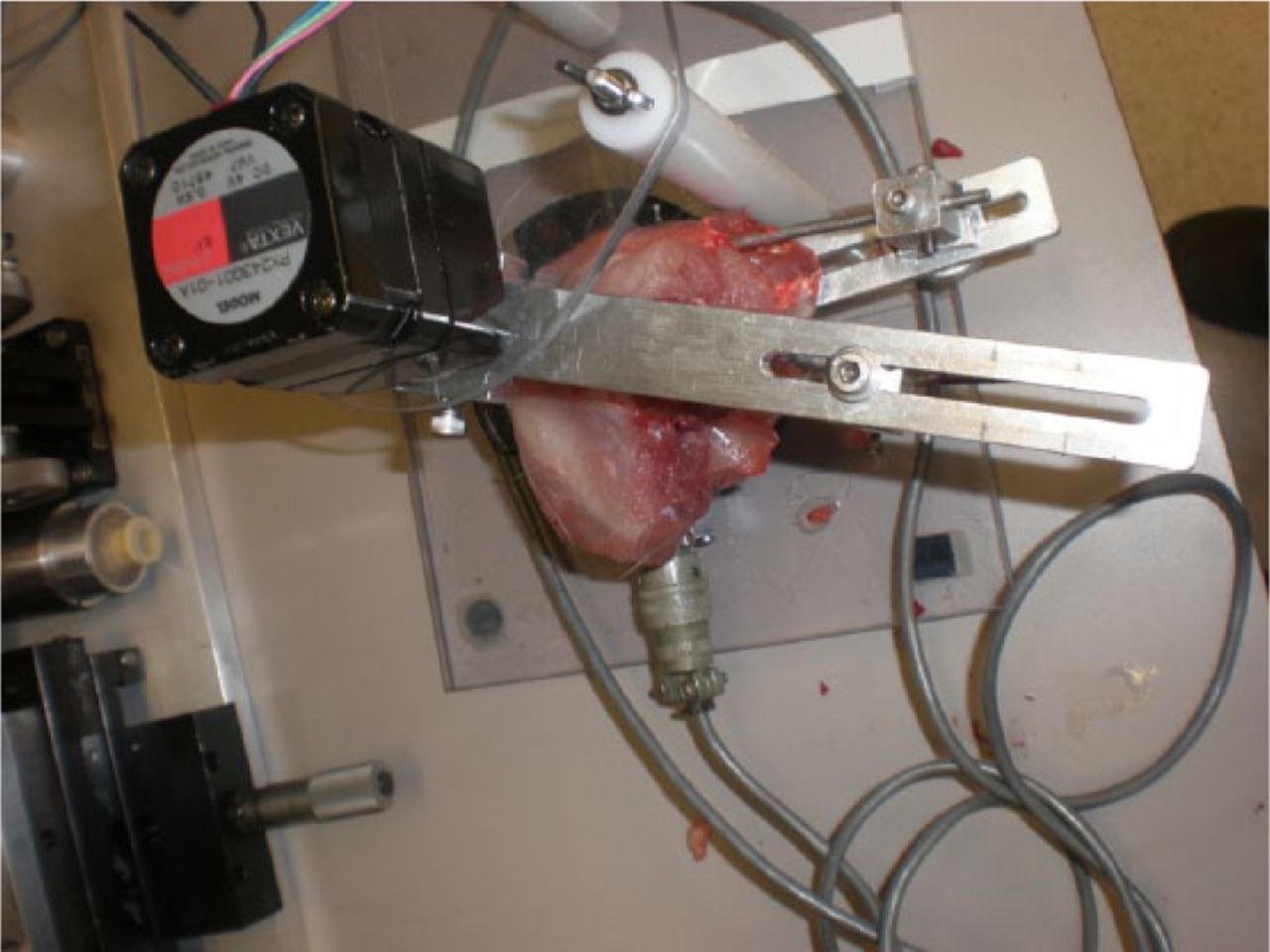
Fig. 3
Photograph of the mechanical testing device. Following mounting of the limb, the joint was extended at 1° per second until 20 Ncm was reached. Fluoroscopic verification that the femoral condyles were centred over the centre of the load cell.
Tissue collection
Following mechanical testing, the posterior capsule of each animal was immediately dissected. Half of the excised tissue was immediately snap frozen in liquid nitrogen, and stored at -80°C for micro-array analysis. The remaining half of the excised tissue was placed in formalin for histological analysis.
Histological analysis
Myofibroblast identification and quantification was completed as previously described.3 Briefly, capsule specimens were stained for α-smooth muscle actin (SMA), and counterstained with haematoxylin to idenfity cell nuclei. Subsequently, three images of joint capsule were taken at random on six serial sections of tissue (18 images total per limb). Myofibroblasts were identified as cell nuclei associated with α-SMA stain. Blood vessels, which also stain positive for α-SMA, were manually excluded based on morphology.
Genetic analysis
mRNA extraction was completed as per previously described protocols.4 The RNA extraction from frozen tissue was performed using a kit from 5 PRIME (Fisher Scientific, Pittsburgh, Pennsylvania). Samples with < 100 ng of RNA or RNA integrity numbers (RIN) of < 6.5 were excluded. Remaining RNA was stored at −80°C until further analysis.
After mRNA extraction, a custom microarray was used to identify significant changes of 384 genes. A cDNA-mediated Annealing, Selection, extension, and Ligation (DASL) assay pool was designed and synthesised through the Illumina, Inc. (San Diego, California) custom DASL service that investigates 384 common rabbit (Oryctolagus cuniculus) mRNA targets. Analysis of mRNA was conducted according to the manufacturer’s instructions for the DASL assay. Quality assessment parameters were determined to be within normal ranges before proceeding to the final data reduction.
Statistical analysis
Total myofibroblast numbers, total cell count numbers, and percentage of myofibroblasts were measured and compared with the non-operative limbs. Direct comparison between experimental groups was done using a two-sample t-test assuming unequal variances. Differences occurring with < 5% likelihood of being due to chance were considered statistically significant (p < 0.05). Data are represented as mean and standard deviation (sd).
The sample size was calculated assuming a 5% type 1 error and 80% power to detect an effect difference of 30° with a sd of 20°. The knee joint flexion angles of the operated limbs in the control animals were measured and compared with the operated limbs in the rosiglitazone-treated animals. Comparison was performed using a two-sample t-test assuming unequal variances. Significance level was set at a p-value of < 0.05. Data are presented as mean and standard deviation.
Gene expression of the control animals and rosiglitazone-treated animals were compared. The Illumina whole-genome genes array was used to assay 384 transcripts, as previously described.4 A total of six samples were run on one array and the samples from the groups were randomly allocated to the arrays. Gene expression data were ‘normalised’ within treatment groups using fastlo, the model-based method of normalisation. Analysis was completed using log base-two expression values and Student’s t-test was used to test for differences between the mean expression levels. Results are presented for p < 0.05.
Results
Biomechanical
Rosiglitazone did not increase the biomechanical effectiveness of a limited capsular release in this model. At the time of contracture release surgery, there was no significant difference in the flexion contracture between the rosiglitazone and control groups (34° sd 12 vs 30° sd 8, respectively; p = 0.32). Following 16 weeks of remobilisation, the difference in flexion contracture between the two groups was also not statistically significant (33° sd 11 vs 37° sd 14, respectively; p = 0.39; Fig. 4). When the overall change in flexion contracture was assessed, as calculated by the difference in flexion contracture intra-operatively and at the final time point, there was no significant difference in the rosiglitazone group compared with controls (-2° vs 7°, respectively; p = 0.059).
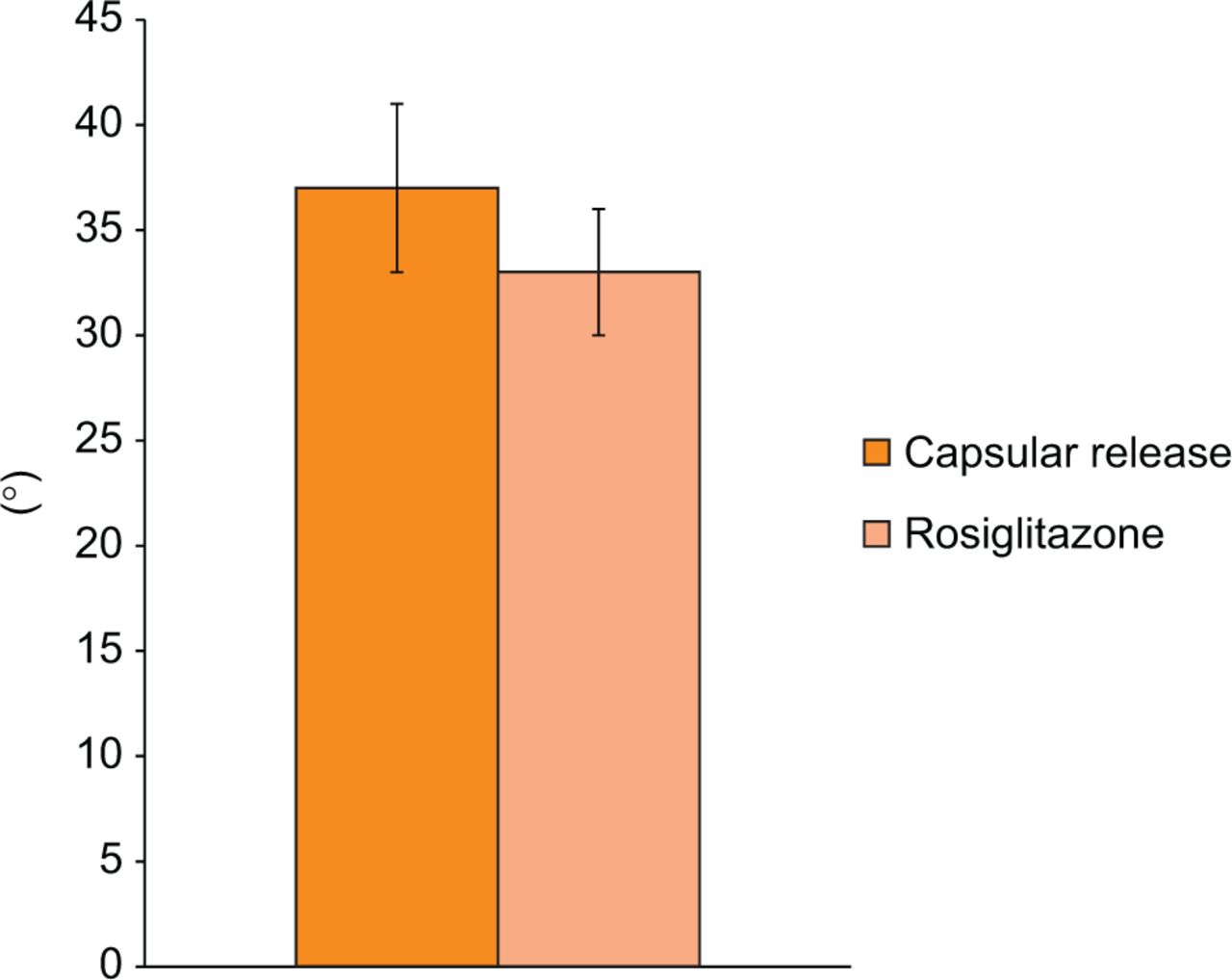
Fig. 4
Graph showing the final flexion contracture. Flexion contracture of the limited capsular release group compared with rosiglitazone group (mean and standard error, p = 0.39).
Histological
There were no significant differences in myofibroblast count, total cell count, and percentage myofibroblasts between animals in the control and rosiglitazone groups. There was a mean of 2% myofibroblasts in the control group and 4% myofibroblasts in the rosiglitazone group at the time of sacrifice. When compared between the groups, this difference was not significant (p = 0.16). The number of myofibroblasts and number of total cells were compared between the groups, and did not demonstrate a significant difference (p = 0.17 and p = 0.13, respectively). Finally, there was no significant difference between the myofibroblast count, total cell count, or percentage of myofibroblasts in the operative versus the non-operative limbs in either group.
Genetic
Of 384 genes tested with the microarray, there was a significant difference detected in ten genes. There was a significant downregulation of defensin NP-3a, interleukin 1 beta (IL-1β), angiotensin-converting enzyme, cyclin A2, serum and glucocorticoid-regulated protein kinase, glutathione s-transferase, and ryk for tyrosine kinase-related protein. There was a significant upregulation of factor XI, BCL2-like 1, and interleukin 6. In addition, there was a nonsignificant downregulation of three genes: collagen type X (fold change: -4.3; p = 0.05), integrin alpha V (fold change = -1.5; p = 0.06), and TGF-β 2 (fold change = -1.3; p = 0.05). There was a nonsignificant upregulation of three genes: fertilin α (fold change = 1.8; p = 0.06), macrophage migration inhibitory factor-related protein 8 (fold change = 1.9; p = 0.06), and apolipoprotein C-IV (fold change = 1.9; p = 0.06) (Table I ).
Table I.
Gene products showing a significant difference in expression in the posterior capsule of rosiglitazone and control animals at the final time point (16 weeks post-remobilisation).
Name | Change in fold | p-value |
---|---|---|
Defensin NP-3a | -4.5 | 0.016 |
Interleukin 1β | -4.0 | 0.024 |
Angiotensin-converting enzyme | -2.0 | 0.027 |
Cyclin A2 | -1.5 | 0.043 |
Serum and glucocorticoid-regulated protein kinase | -1.5 | 0.042 |
Glutathione S-Transferase | -1.2 | 0.022 |
Ryk for Tyrosine kinase-related protein | -1.1 | 0.026 |
Factor XI | 1.2 | 0.045 |
BCL2-like 1 | 3.3 | 0.021 |
Interleukin 6 | 7.1 | 0.043 |
Gene products showing a (0.05 < p < 0.065) a significant difference | ||
Collagen type X | -4.3 | 0.052 |
Integrin Alpha V | -1.5 | 0.058 |
TGF-β 2 | -1.3 | 0.054 |
Fertilin α | 1.8 | 0.063 |
Macrophage migration inhibitory factor | 1.9 | 0.064 |
Apolipoprotein C | 1.9 | 0.055 |
The bioinformatics analysis generated 15 pathways that were significantly implicated (FDR filter 0.05) (Table II). Pigment epithelium-derived factor (PEDF) signalling, CD40 signalling, the TH17 cell differentiation pathway, and the macrophage migration inhibitory factor (MIF) pathway were pathways of most significance to fibrosis formation.
Table II.
Pathways that were significantly involved, when comparing rosiglitazone with control groups.
Pathway | p-value |
---|---|
Development: PEDF signalling | 1.0 × 10-5 |
Immune response: role of HMGB1 in dendritic cell maturation and migration | 2.8 × 10-4 |
Immune response: TH17 cell differentiation | 4.7 × 10-4 |
Immune response: HMGB1/TLR signalling pathway | 4.7 × 10-4 |
Immune response: MIF in innate immunity response | 6.1 × 10-4 |
Immune response: HMGB1 release from the cell | 6.4 × 10-4 |
Immune response: IL-1 signalling pathway | 7.4 × 10-4 |
Immune response: Inhibitory action of lipoxins on pro-inflammatory TNF-alpha signalling | 8.5 × 10-4 |
Immune response: Histamine signalling in dendritic cells | 9.6 × 10-4 |
Immune response: HMGB1/RAGE signalling pathway | 1.1 × 10-3 |
Immune response: TREM1 signalling pathway | 1.3 × 10-3 |
Immune response: IL-17 signalling pathways | 1.4 × 10-3 |
Transcription role of VDR in regulation of genes involved in osteoporosis | 1.4 × 10-3 |
Immune response IL-15 signalling | 1.6 × 10-3 |
Immune response: CD40 signalling | 1.6 × 10-3 |
Discussion
There is ongoing interest in the biomechanical, histological and genetic processes of joint contractures. Our group established a model that includes a surgical capsular release at the time of remobilisation of the animals. This model has the additional advantage of allowing therapeutic agents to be carefully administered at the time of this intervention. In this experiment, we sought to determine the effectiveness of rosiglitazone in reducing joint contracture by assessing: the biomechanical effects of rosiglitazone by measuring the immediate post-operative and final joint contracture following 16 weeks of treatment; the histological effects of rosiglitazone by measuring the myofibroblast count in the capsular tissue; and the genetic effects of rosiglitazone by using a rabbit gene microarray.
In this model, there was no significant difference between the animals receiving rosiglitazone versus placebo, based on flexion contracture or histology. This is an unexpected finding given the current literature supporting the role of PPAR-γ agonists in TGF-β modulation and fibrosis. This may be related to low effective concentrations in the joint, or inadequate drug dosage.
The genetic analysis demonstrated ten genes and 15 pathways that were significantly modulated in the rosiglitazone group compared with the control group. Previous studies have demonstrated that TGF-β drives fibrosis through three main processes: inflammatory cell proliferation and migration, epithelial-mesenchymal transition and matrix production.22-24 Four specific groups of pathways and genes warrant further discussion, for their implications in these processes:
-
− The PEDF signalling pathway was significantly modulated in the rosiglitazone group in this study. PEDF has been demonstrated to be antifibrogenic in pulmonary, renal and hepatic fibrosis.25 PEDF signalling leads to a decrease in TGF-β expression.26 Much of this anti-TGF-β and antifibrogenic effect is modulated by PPAR-γ.27,28 Modulation of this pathway by rosiglitazone was confirmed in this study.
-
− Another significant signalling pathway that was modulated in the rosiglitazone group in this study was the CD40 pathway. This pathway is the subject of significant ongoing research in fibrosis development. PPAR-γ agonists have been shown to decrease CD40 signalling in fibroblasts.29,30 CD40 has been implicated as a profibrogenic molecule, with increased CD40 expression in patients with systemic sclerosis, as well as lung and peritoneal fibrosis.31-35 Furthermore, decreasing CD40 activity has been demonstrated to decrease fibrosis.36,37 Modulation of the CD40 pathway is another possible therapeutic effect of rosiglitazone demonstrated in this study.
-
− The TH17 cell differentiation pathway and the IL-17 signalling pathways were also modulated in this study. These pathways are intimately associated, as TH17 cells are lymphocytes that secrete IL-17. Previous work has demonstrated that the PPAR-γ agonists inhibit TH17 differentiation and TH17 responses.38,39 Although the exact fibrotic responses to IL-17 and TH17 are still being elucidated, the preponderance of evidence would indicate an antifibrogenic activity of PPAR-γ agonists. In vitro studies on human cells have demonstrated that IL-17 increases the expression of proinflammatory cytokines and enhances fibroblast proliferation.40,41 Although further work on the exact role of IL-17 and TH17 in the role of fibrosis is required, the modulation of this pathway by rosiglitazone would indicate an antifibrogenic effect.
-
− A final pathway that demonstrated a significant modulation by rosiglitazone was the MIF pathway. In addition, MIF-related protein-8 demonstrated upregulation (fold change: 1.9, p = 0.064). MIF has been shown to increase IL-1β expression in fibroblasts.42 It has also been implicated in increasing inflammation in rheumatoid arthritis. Finally, studies of kidney and liver fibrosis have demonstrated increased MIF expression.43,44 Modulation of this pathway by rosiglitazone has the potential to modulate fibrosis in this setting.
A limitation of this study is that joint angle measurements made at the time of the operation were done using fluoroscopic imaging at the time of operation, with gentle manual extension applied by the surgeon’s hand, rather than using the custom testing device. While this allows a gross assessment of movement, it lacks the precision of the joint angle measuring device that is used at final follow-up. Unfortunately, the joint angle measuring device requires sacrifice for testing, limiting the ability to use it at the time of the release operation. This reduces the depth of analysis possible on the post-operative flexion contracture measurements. Finally, no measurements of serum or joint concentration of rosiglitazone were performed to assess the effective drug levels.
Overall, rosiglitazone had no statistically significant effect on biomechanical or histological properties, but did impact the genetic expression of the posterior capsular tissue in a rabbit model of contracture, with ten gene products and 17 pathways demonstrating significant modulation. The effects of rosiglitazone on these pathways have the potential to ameliorate the formation of fibrosis. Further work, including analysis of the genetic pathways, more precise drug delivery, and different time points of analysis may provide more insight into the possible therapeutic role of rosiglitazone in this disease process.
Funding Statement:
B. F. Morrey reports funding received from Zimmer, Stryker, DJO and Tenex Health, none of which is related to this article.
ICMJE conflict of interest:
None declared.
References
1. Brinsden MD , CarrAJ, ReesJL. Post-traumatic flexion contractures of the elbow: operative treatment via the limited lateral approach. J Orthop Surg Res2008;3:39.CrossrefPubMed Google Scholar
2. Efird W , KellamP, YeazellS, WeinholdP, DahnersLE. An evaluation of prophylactic treatments to prevent post traumatic joint stiffness. J Orthop Res2014;32:1520-1524.CrossrefPubMed Google Scholar
3. Abdel MP , MorreyME, BarlowJD, et al.. Myofibroblast cells are preferentially expressed early in a rabbit model of joint contracture. J Orthop Res2012;30:713-719.CrossrefPubMed Google Scholar
4. Abdel MP , MorreyME, GrillDE, et al.. Effects of joint contracture on the contralateral unoperated limb in a rabbit knee contracture model: a biomechanical and genetic study. J Orthop Res2012;30:1581-1585.CrossrefPubMed Google Scholar
5. Hildebrand KA , SutherlandC, ZhangM. Rabbit knee model of post-traumatic joint contractures: the long-term natural history of motion loss and myofibroblasts. J Orthop Res2004;22:313-320.CrossrefPubMed Google Scholar
6. Hildebrand KA , ZhangM, HartDA. Joint capsule matrix turnover in a rabbit model of chronic joint contractures: correlation with human contractures. J Orthop Res2006;24:1036-1043.CrossrefPubMed Google Scholar
7. Nesterenko S , MorreyME, AbdelMP, et al.. New rabbit knee model of posttraumatic joint contracture: indirect capsular damage induces a severe contracture. J Orthop Res2009;27:1028-1032.CrossrefPubMed Google Scholar
8. Dávila SA , Johnston-JonesK. Managing the stiff elbow: operative, nonoperative, and postoperative techniques. J Hand Ther2006;19:268-281.CrossrefPubMed Google Scholar
9. Morrey BF . Posttraumatic stiffness: distraction arthroplasty. Orthopedics1992;15:863-869.CrossrefPubMed Google Scholar
10. Morrey BF . Surgical treatment of extraarticular elbow contracture. Clin Orthop Relat Res2000;370:57-64.CrossrefPubMed Google Scholar
11. Morrey BF . The posttraumatic stiff elbow. Clin Orthop Relat Res2005;431:26-35.CrossrefPubMed Google Scholar
12. Lindenhovius AL , vande, LuijtgaardenK, RingD, JupiterJ. Open elbow contracture release: postoperative management with and without continuous passive motion. J Hand Surg Am2009;34:858-865.CrossrefPubMed Google Scholar
13. Stans AA , MaritzNG, O’DriscollSW, MorreyBF. Operative treatment of elbow contracture in patients twenty-one years of age or younger. J Bone Joint Surg [Am]2002;84-A:382-387. Google Scholar
14. Barlow JD , HartzlerRU, AbdelMP, et al.. Surgical capsular release reduces flexion contracture in a rabbit model of arthrofibrosis. J Orthop Res2013;31:1529-1532.CrossrefPubMed Google Scholar
15. Sgonc R , WickG. Pro- and anti-fibrotic effects of TGF-beta in scleroderma. Rheumatology (Oxford)2008;(47Suppl 5):v5-v7.CrossrefPubMed Google Scholar
16. Abdel MP , MorreyME, BarlowJD, et al.. Intra-articular decorin influences the fibrosis genetic expression profile in a rabbit model of joint contracture. Bone Joint Res2014;3:82-88.CrossrefPubMed Google Scholar
17. Sime PJ . The antifibrogenic potential of PPARgamma ligands in pulmonary fibrosis. J Investig Med2008;56:534-538.CrossrefPubMed Google Scholar
18. Wynn TA . Cellular and molecular mechanisms of fibrosis. J Pathol2008;214:199-210.CrossrefPubMed Google Scholar
19. Lakota K , WeiJ, CarnsM, et al.. Levels of adiponectin, a marker for PPAR-gamma activity, correlate with skin fibrosis in systemic sclerosis: potential utility as biomarker?Arthritis Res Ther2012;14:R102.CrossrefPubMed Google Scholar
20. Pan HW , XuJT, ChenJS. Pioglitazone inhibits TGFβ induced keratocyte transformation to myofibroblast and extracellular matrix production. Mol Biol Rep2011;38:4501-4508.CrossrefPubMed Google Scholar
21. Wu M , MelichianDS, ChangE, et al.. Rosiglitazone abrogates bleomycin-induced scleroderma and blocks profibrotic responses through peroxisome proliferator-activated receptor-gamma. Am J Pathol2009;174:519-533.CrossrefPubMed Google Scholar
22. Bhattacharyya S , WeiJ, TourtellotteWG, et al.. Fibrosis in systemic sclerosis: common and unique pathobiology. Fibrogenesis Tissue Repair2012;5(Suppl 1):S18.CrossrefPubMed Google Scholar
23. Bi WR , YangCQ, ShiQ. Transforming growth factor-β1 induced epithelial-mesenchymal transition in hepatic fibrosis. Hepatogastroenterology2012;59:1960-1963.CrossrefPubMed Google Scholar
24. Edgley AJ , KrumH, KellyDJ. Targeting fibrosis for the treatment of heart failure: a role for transforming growth factor-β. Cardiovasc Ther2012;30:e30-e40.CrossrefPubMed Google Scholar
25. Wang JJ , ZhangSX, LuK, et al.. Decreased expression of pigment epithelium-derived factor is involved in the pathogenesis of diabetic nephropathy. Diabetes2005;54:243-250.CrossrefPubMed Google Scholar
26. Ho TC , ChenSL, ShihSC, et al.. Pigment epithelium-derived factor is an intrinsic antifibrosis factor targeting hepatic stellate cells. Am J Pathol2010;177:1798-1811.CrossrefPubMed Google Scholar
27. Ho TC , ChenSL, YangYC, et al.. PEDF induces p53-mediated apoptosis through PPAR gamma signaling in human umbilical vein endothelial cells. Cardiovasc Res2007;76:213-223.CrossrefPubMed Google Scholar
28. Ho TC , YangYC, ChenSL, et al.. Pigment epithelium-derived factor induces THP-1 macrophage apoptosis and necrosis by the induction of the peroxisome proliferator-activated receptor gamma. Mol Immunol2008;45:898-909.CrossrefPubMed Google Scholar
29. Zhang YF , YangX, ZhangYJ, et al.. Peroxisome proliferator-activated receptor-gamma is expressed by rat peritoneal mesothelial cells: its potential role in peritoneal cavity local defense. Am J Nephrol2006;26:602-611.CrossrefPubMed Google Scholar
30. Oxer DS , GodoyLC, BorbaE, et al.. PPARγ expression is increased in systemic lupus erythematosus patients and represses CD40/CD40L signaling pathway. Lupus2011;20:575-587.CrossrefPubMed Google Scholar
31. Atamas SP , LuzinaIG, DaiH, WiltSG, WhiteB. Synergy between CD40 ligation and IL-4 on fibroblast proliferation involves IL-4 receptor signaling. J Immunol2002;168:1139-1145.CrossrefPubMed Google Scholar
32. Fukasawa C , KawaguchiY, HarigaiM, et al.. Increased CD40 expression in skin fibroblasts from patients with systemic sclerosis (SSc): role of CD40-CD154 in the phenotype of SSc fibroblasts. Eur J Immunol2003;33:2792-2800.CrossrefPubMed Google Scholar
33. Kawai M , MasudaA, KuwanaM. A CD40-CD154 interaction in tissue fibrosis. Arthritis Rheum2008;58:3562-3573.CrossrefPubMed Google Scholar
34. Mehling A , LoserK, VargaG, et al.. Overexpression of CD40 ligand in murine epidermis results in chronic skin inflammation and systemic autoimmunity. J Exp Med2001;194:615-628.CrossrefPubMed Google Scholar
35. Zhang-Hoover J , SuttonA, Stein-StreileinJ. CD40/CD40 ligand interactions are critical for elicitation of autoimmune-mediated fibrosis in the lung. J Immunol2001;166:3556-3563.CrossrefPubMed Google Scholar
36. Adawi A , ZhangY, BaggsR, et al.. Blockade of CD40-CD40 ligand interactions protects against radiation-induced pulmonary inflammation and fibrosis. Clin Immunol Immunopathol1998;89:222-230.CrossrefPubMed Google Scholar
37. Komura K , FujimotoM, YanabaK, et al.. Blockade of CD40/CD40 ligand interactions attenuates skin fibrosis and autoimmunity in the tight-skin mouse. Ann Rheum Dis2008;67:867-872.CrossrefPubMed Google Scholar
38. Klotz L , BurgdorfS, DaniI, et al.. The nuclear receptor PPAR gamma selectively inhibits Th17 differentiation in a T cell-intrinsic fashion and suppresses CNS autoimmunityJ Exp Med2009;206:2079-2089.CrossrefPubMed Google Scholar
39. Kanakasabai S , ChearwaeW, WallineCC, et al.. Peroxisome proliferator-activated receptor delta agonists inhibit T helper type 1 (Th1) and Th17 responses in experimental allergic encephalomyelitis. Immunology2010;130:572-588.CrossrefPubMed Google Scholar
40. Kurasawa K , HiroseK, SanoH, et al.. Increased interleukin-17 production in patients with systemic sclerosis. Arthritis Rheum2000;43:2455-2463.CrossrefPubMed Google Scholar
41. Fossiez F , DjossouO, ChomaratP, et al.. T cell interleukin-17 induces stromal cells to produce proinflammatory and hematopoietic cytokines. J Exp Med1996;183:2593-2603.CrossrefPubMed Google Scholar
42. Onodera S , NishihiraJ, KoyamaY, et al.. Macrophage migration inhibitory factor up-regulates the expression of interleukin-8 messenger RNA in synovial fibroblasts of rheumatoid arthritis patients: common transcriptional regulatory mechanism between interleukin-8 and interleukin-1beta. Arthritis Rheum2004;50:1437-1447.CrossrefPubMed Google Scholar
43. Aeberli D , LeechM, MorandEF. Macrophage migration inhibitory factor and glucocorticoid sensitivity. Rheumatology (Oxford)2006;45:937-943.CrossrefPubMed Google Scholar
44. Tripathi G , BorkarM, AkhterA, et al.. Association of proinflammatory cytokines with end stage renal disease. Cytokine2010;50:278-283.CrossrefPubMed Google Scholar