Abstract
Objectives
Traumatic brachial plexus injury causes severe functional impairment of the arm. Elbow flexion is often affected. Nerve surgery or tendon transfers provide the only means to obtain improved elbow flexion. Unfortunately, the functionality of the arm often remains insufficient. Stem cell therapy could potentially improve muscle strength and avoid muscle-tendon transfer. This pilot study assesses the safety and regenerative potential of autologous bone marrow-derived mononuclear cell injection in partially denervated biceps.
Methods
Nine brachial plexus patients with insufficient elbow flexion (i.e., partial denervation) received intramuscular escalating doses of autologous bone marrow-derived mononuclear cells, combined with tendon transfers. Effect parameters included biceps biopsies, motor unit analysis on needle electromyography and computerised muscle tomography, before and after cell therapy.
Results
No adverse effects in vital signs, bone marrow aspiration sites, injection sites, or surgical wound were seen. After cell therapy there was a 52% decrease in muscle fibrosis (p = 0.01), an 80% increase in myofibre diameter (p = 0.007), a 50% increase in satellite cells (p = 0.045) and an 83% increase in capillary-to-myofibre ratio (p < 0.001) was shown. CT analysis demonstrated a 48% decrease in mean muscle density (p = 0.009). Motor unit analysis showed a mean increase of 36% in motor unit amplitude (p = 0.045), 22% increase in duration (p = 0.005) and 29% increase in number of phases (p = 0.002).
Conclusions
Mononuclear cell injection in partly denervated muscle of brachial plexus patients is safe. The results suggest enhanced muscle reinnervation and regeneration.
Cite this article: Bone Joint Res 2014;3:38–47.
Article focus
Is autologous bone marrow-derived mononuclear cell injection in partly denervated muscle of traumatic brachial plexus patients safe?
Can mononuclear cell injection cause reinnervation and regeneration of the denervated muscle?
Key messages
Following autologous bone marrow-derived mononuclear cell injection no adverse effects were seen
After cell transplantation quantitative needle electromyography improved, suggestive for muscle reinnervation
Computerised muscle tomography and histological assessment showed muscle improvement, suggestive for myogenic regeneration
Strengths and limitations
Strength: a profound pilot study using blinded histology, quantitative needle electromyography and computerised muscle tomography as objective effect parameters
Limitations: the small number of patients and the lack of a control patient group. The promising results of the study require confirmation in a larger controlled clinical study
Introduction
Brachial plexus (BP) injury is often a result of a high-energy road traffic accident in young adults.1,2 The upper BP (C5-C6) is commonly affected, resulting in paresis of shoulder function and elbow flexion.1-4 Nerve surgery is aimed at restoring innervation of the bicepsmuscle, but often a deficit in the functionality of the arm remains. In that case, secondary surgery can improve arm function by transfer of the flexor-pronator group of the forearm (Steindler procedure) for active elbow flexion.5-10 However, neither nerve surgery nor tendon transfers come close to restoring normal elbow flexion.5
Any procedure that improves elbow flexion without sacrificing the function of transferred donor muscles will have advantages for the patient. One approach is to improve the regenerative capacity of the partially denervated muscles. Denervation causes loss of contractile force and muscle atrophy. If innervation is restored quickly, good restorative ability exists. Conversely, prolonged interruption of innnervation results in irreversible muscle atrophy, interstitial fibrosis and muscle fattening.11-14 Since regeneration of denervated muscles not only depends on nerve supply, but also on its regenerative capability, the muscle can be a target organ for cell therapy.
Muscle satellite cells are responsible for repair and maintenance of skeletal muscles; they are the main source of new myofibres. Myogenic precursor cells proliferate, fuse and form new myofibres in response to muscle damage.15 Exhaustion of the pool of available satellite cells may contribute to poor functional recovery of long-term denervated muscle.16,17 A progressive and rapid decrease in the capillary-to-muscle fibre ratio occurs, further limiting regeneration.18
Transplantation of primary satellite cells has been shown to improve the properties of reinnervated skeletal muscles in rabbits.19 However, poor cellular survival and limited cell dissemination hampers successful satellite cell transplantation. Furthermore, only few cells fuse with host fibres. This suggests that a subpopulation of myogenic cells (i.e. stem cells) may be optimally suited for transplantation.20,21 Bone marrow (BM)-derived cells migrate to the site of muscle injury and contribute to the satellite cell pool.22-24
The injection of autologous BM-derived mononuclear cells (MNCs) has been applied in clinical studies focusing on the muscles of the heart and leg.25-28
The primary objective was to assess the safety of autologous BM-derived MNC injection in partially denervated muscles of BP patients. The secondary objective was to obtain a first estimate on regenerative potential of injected BM-derived MNCs in partially denervated muscles.
Patients and Methods
Study design
This was a prospective study on autologous BM-derived MNC therapy for partially denervated biceps muscles of BP patients, with focus on safety and the effect on muscle. Three escalating doses were evaluated in three groups, each comprising three patients. The MNC dose was equivalent to 50% (group A), 100% (group B), and 200% (group C) of the cell dose in a former study at our institution.25
The protocol was approved by the medical ethics committee of Leiden University Medical Center. The Declaration of Helsinki protocols were followed and patients gave written, informed consent. The clinical trial was registered under ClinicalTrials.gov identifier: NCT00755586.
Patients
Nine adult traumatic BP patients with partial denervation of the biceps muscle (Medical Research Council (MRC) grades between 1 and 3)29 were included. All patients were at an end-stage of functional results (i.e. ≥ 2 years after trauma or nerve surgery). Patients with a complete paralysis of the biceps muscle were excluded as they were assumed to have no regenerative capacity (MRC grade 0). This was corroborated by the absence of motor unit potentials (MUPs) during needle electromyography (EMG). Other exclusion criteria were a history of central or other peripheral neurological disorders, humeral fractures, and contraindication for BM harvesting (such as bleeding diathesis or an international normalised ratio (INR) > 2).
BM aspiration and MNC separation
BM was aspirated from one location at the posterior iliac crest. The procedure was performed under local (group A) or general anaesthesia (groups B and C). Due to ethical considerations associated with the use of general anaesthesia, in groups B and C the MNC injection was combined with a Steindler procedure,30 which was considered the standard surgical procedure in patients with an elbow flexion deficiency.
BM was separated as previously described.25,28 The isolated MNCs were concentrated in a volume of 10 ml. The isolation and concentration procedure was performed in a certified clean-room facility according to good manufacturing practice (GMP).25,28 Further details of the methods are provided as supplementary material.
MNC injection
BM-derived MNCs were injected at 20 sites at the maximum palpable thickness of the biceps and at a standard injection depth of 0.5 cm. A total volume of 10 mL was injected. Further details of the methods are provided as supplementary material.
Safety
In order to assure safety, the absence of adverse events and muscle fibrosis was documented before proceeding to the next dose level. Vital signs were checked and the BM aspiration, MNC injection and surgical wound site were examined during a 24-hour hospital admission and at three and six months after cell therapy. The safety of MNC injection at muscle level was assessed by CT analysis and histology.
Evaluation of patients
All patients were evaluated pre-operatively and at three and six months post-operatively using clinical scores, CT analysis and EMG. Histology of the biceps muscle was undertaken pre-operatively and at three months post-operatively. Before analysis, all images were blinded for date and patients characteristics.
Clinical functionality
Active and passive range of movement (ROM) of the injured elbow was measured using a handheld goniometer. Force measurement of the injured biceps muscle was assessed using the MRC motor scale. Pain at rest was scored using a visual analogue scale (VAS) (0 - no pain to 10 - extreme pain). Finally, the Disabilities of the Arm, Shoulder, And Hand (DASH) questionnaire31 and the Short-Form Health Survey (SF-) 3632 were used to measure quality of life and functional outcome. To compare the DASH score with the SF-36 we calibrated the score to a 100-point metric score from 0 (worst health) to 100 (best health). The SF-36 score ranges from 0 (worst health) to 100 (best health).
CT scan
Patients were scanned using the Aquilion 64-slice CT scanner (Toshiba Medical Systems, Otawara, Japan). A total of 50 consecutive 1.0-mm multiplane reconstruction (MPR) images were computed in the coronal plane perpendicular to the main axis of the humerus. The MPR images were analysed and the mean muscle density (MMD) was calculated.33The MMD of the injured (injected) biceps muscle was compared with control muscles (the injured (non-injected) triceps muscle and non-injured (non-injected) contralateral biceps muscle). Further details of the methods are provided as supplementary material.
Quantitative needle EMG
Quantitative needle EMG was performed using disposable EMG needle electrodes with a Medelec Synergy EMG system (Oxford Instruments, Oxford, United Kingdom). EMG of the injuredbiceps muscle was compared with that of control muscles (the injured (non-injected) brachialis muscle and non-injured (non-injected) contralateral biceps muscle). Since the mean number of MUPs of the triceps muscle was too low (≤ 2), the brachialis muscle was selected as a control. The multi-MUP analysis was performed using Medelec Synergy software version 11 (Oxford Instruments).34 Further details of the methods are provided as supplementary material.
Histochemistry and immunohistochemistry of muscle biopsy
A biopsy of 0.5 cm × 0.5 cm × 0.5 cm of the injured biceps musclewas taken at the site of the MNC injection.35 It was immediately fixed in 4% buffered formalin and embedded in paraffin. Tissue sections of 5-µ thickness were cut, deparaffinised and rehydrated for Masson’s trichrome staining, routine haematoxylin and eosin (H& E) staining and immunohistochemistry to evaluate muscle, vascular and neural regeneration. For immunohistochemistry Pax7, CD56 and von Willebrand Factor (vWF) were used. Further details of the methods are provided as supplementary material.
Muscle analysis
Muscle sections were analysed using a Zeiss image analysis system (KS400; Zeiss, Sliedrecht, The Netherlands) coupled to a camera (DXC-950P; Sony, Amsterdam, The Netherlands) and ImageJ software v1.38X (National Institutes of Health, Bethesda, Maryland). From each stained section, random images were selected, analysing at least 200 fibres. Further details of the methods are provided as supplementary material.
Statistical analysis
All tests were two-tailed and p-values < 0.05 were considered significant. SPSS v20.0 (SPSS Inc., Chicago, Illinois) was used to perform the analyses. Baseline characteristics are presented as mean and range. Data from muscle biopsies, EMG, CT, and clinical function are presented as mean and standard deviation (sd). Elbow flexion strength using the MRC motor scale is displayed as median and range. A paired t-test or one-way analysis of variance (ANOVA) was used to analyse the muscle biopsy parameters. A mixed model analysis was used to analyse EMG, CT and clinical functionality parameters. Patients were included as random effects on each outcome parameter. In order to determine changes in outcome at follow-up, the variable ‘time’ was included as fixed effect. In order to determine any dose–effect response between group A, B and C, the variable ‘group’ was also included in the mixed model as a fixed effect.
Results
Baseline patient and injected MNC characteristics are shown in Table I. During hospital stay and follow-up, no adverse events in vital signs (blood pressure, pulse rate, temperature), BM aspiration site, injection sites or surgical wound (haematoma, infection) were observed. Post-operatively, the mean decrease in haemoglobin (Hb) concentration was 0.2 mmol/l (group A), 1.0 mmol/l (group B), and 1.8 mmol/l (group C). Hb concentration was normalised in all patients six months after BM aspiration.
Table I
Baseline patient and injected mononuclear cell characteristics in groups A, B and C (MRC, Medical Research Council)
Group A (n = 3) | Group B (n = 3) | Group C (n = 3) | |
---|---|---|---|
Mean age (yrs) (range) | 33 (20 to 45) | 30 (25 to 33) | 23 (20 to 28) |
Male (n, %) | 3 (100) | 3 (100) | 3 (100) |
Brachial plexus lesion type (n, %) | |||
C5-C6 | 1 (33) | 1 (33) | 0 |
C5-C7 | 0 | 2 (67) | 3 (100) |
C5-Th1 | 2 (67) | 0 | 0 |
Mean time after trauma (yrs) (range) | 7.4 (2 to 17) | 8.0 (3 to 17) | 2.9 (2 to 3) |
Neurosurgery (n, %) | 3 (100) | 2 (67) | 3 (100) |
Time after neurosurgery (yrs) (range) | 7.0 (2 to 17) | 4 and 16 | 2.6 (2 to 3) |
Median elbow flexion strength (MRC scale) (range) | 3 (3 to 3) | 2 (2 to 3) | 2 (2 to 3) |
Mean active range of elbow flexion (°) (range) | 65 (25 to 100) | 12 (0 to 35) | 30 (0 to 90) |
Mean injected mononuclear cells (×108 cells) (range) | 0.89 (0.6 to 1.3) | 4 9* | 8* |
CD 34+ (×106 cells) (range) | 2.5 (0.5 to 5) | 10.8 (7 to 16) | 23.6 (15 to 32) |
CD 34- (×106 cells) (range) | 86.5 (57 to 127) | 389.2 (384 to 393) | 776.4 (769 to 785) |
-
*No ranges necessary as more BM under anaesthesia was retracted and exactly 4 x 10 8 injected.
In order to assess the safety of MNC injection at a local muscular level, quantitative CT analysis and histology were used. With quantitative CT analysis, the MMD of the injured biceps muscle was compared with injured and non-injured control muscles. A significant decrease in the MMD of the injured biceps muscles in the total patient group was observed (p = 0.03). The decrease was 32% at three months (p = 0.04) and 48% at six months follow-up (p = 0.009). No dose effect was observed. The non-injured contralateral biceps and the injured (non-injected) triceps muscle showed no significant change in MMD (Fig. 1).
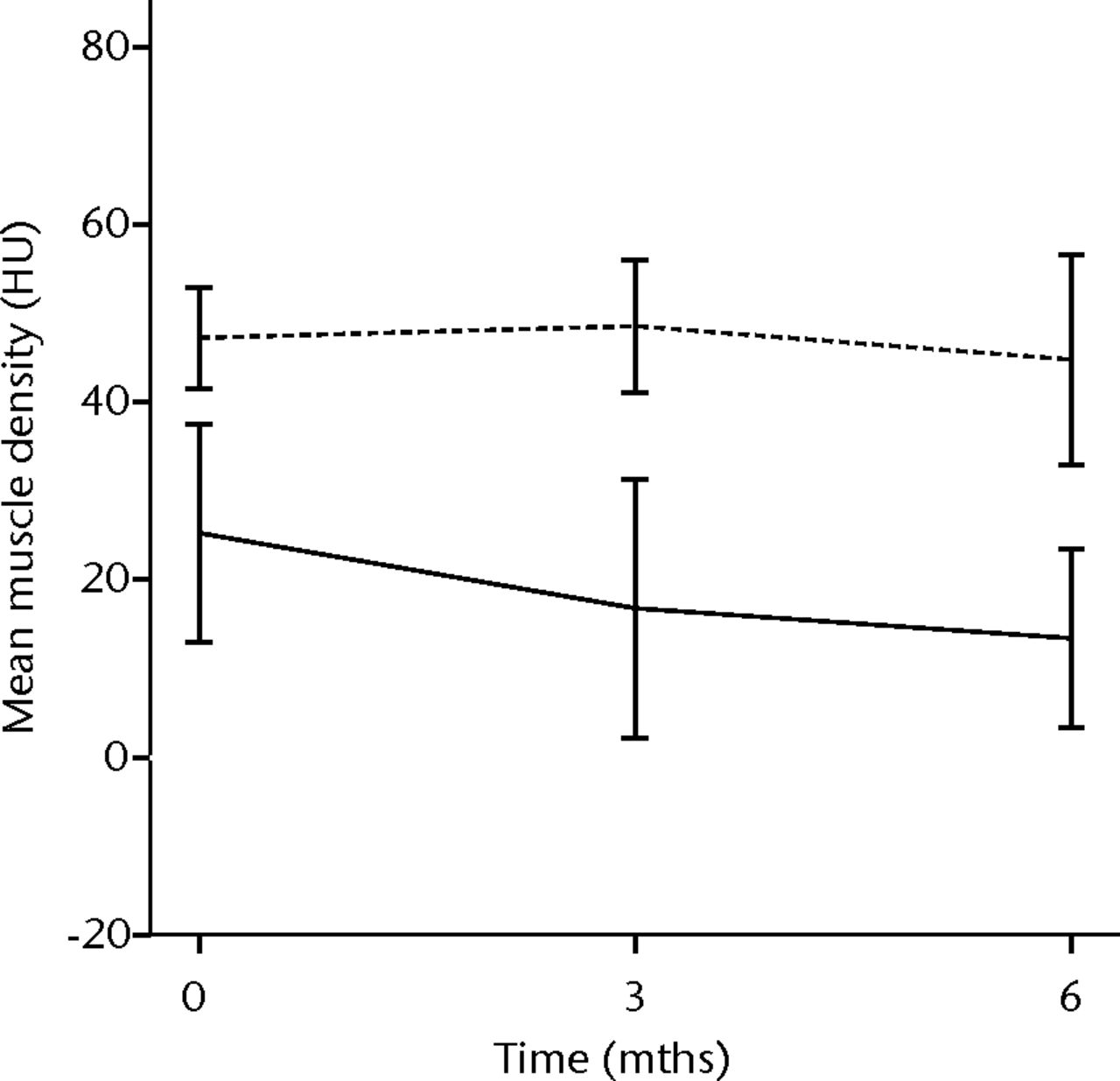
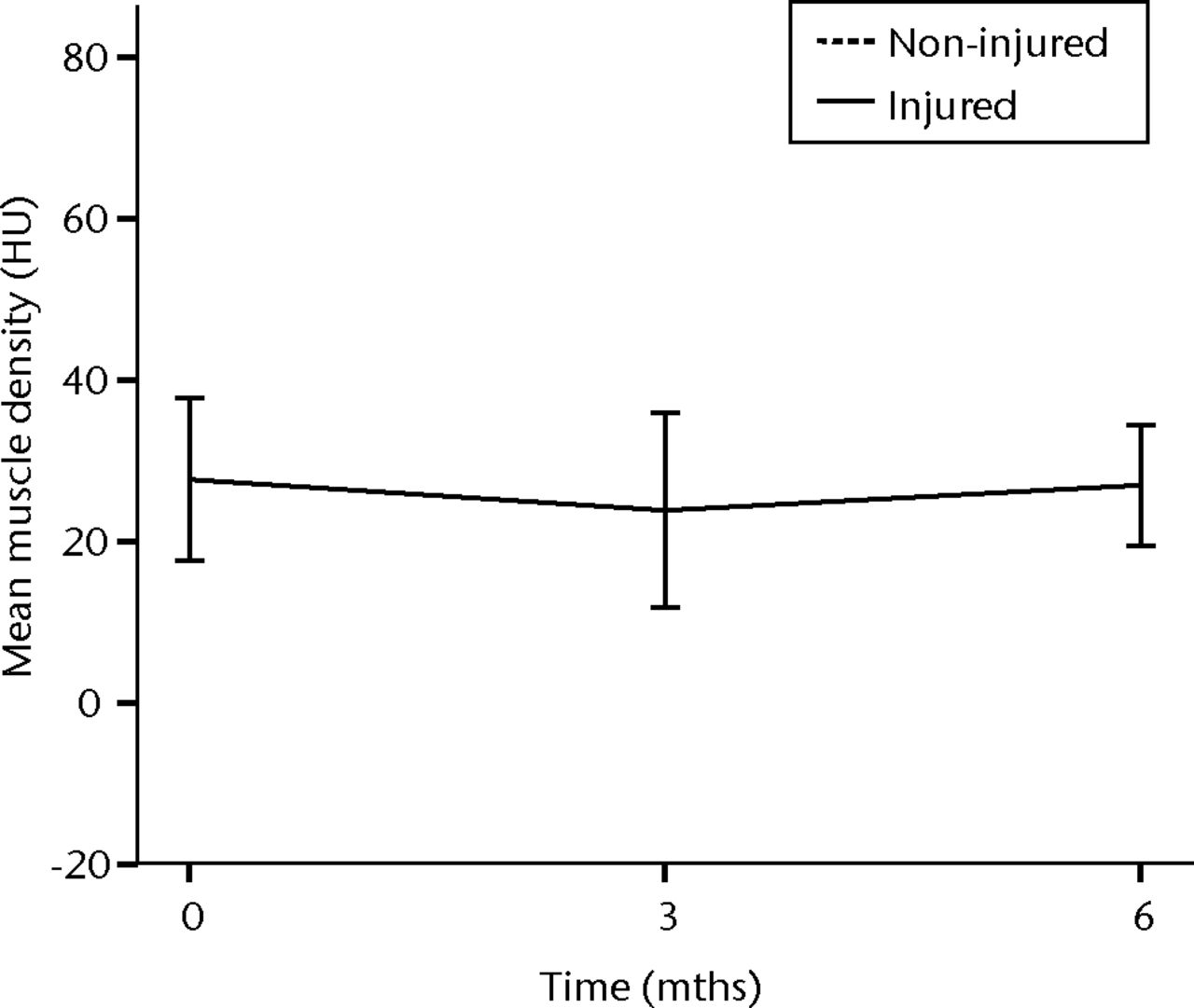
Figs. 1a - 1b
Graphs showing the mean muscle density (MMD) of the injured and non-injured biceps a) and triceps b) muscle pre-transplantation and at three and six months follow-up for the total patient group. Error bars denote 95% confidence interval (HU, Hounsfield unit).
A Masson’s Trichrome staining was performed to analyse the possible formation of muscle fibrosis after cell injection. Compared with the pre-injection biopsy, a decrease of 52% in mean areas of fibrosis of the injured biceps muscle was measured at three months after cell injection in the total patient group (p= 0.01). No dose effect was observed (Fig. 2).
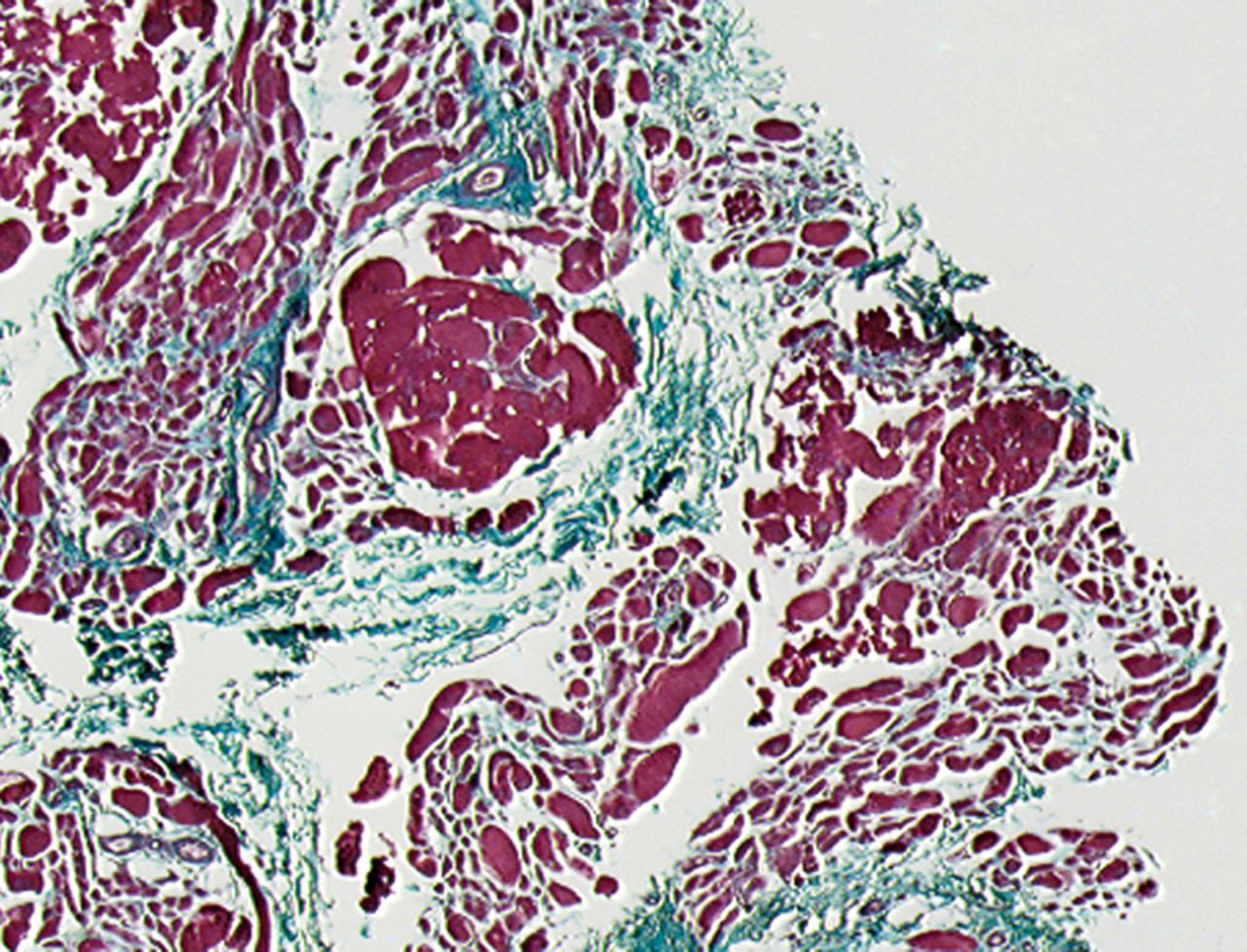
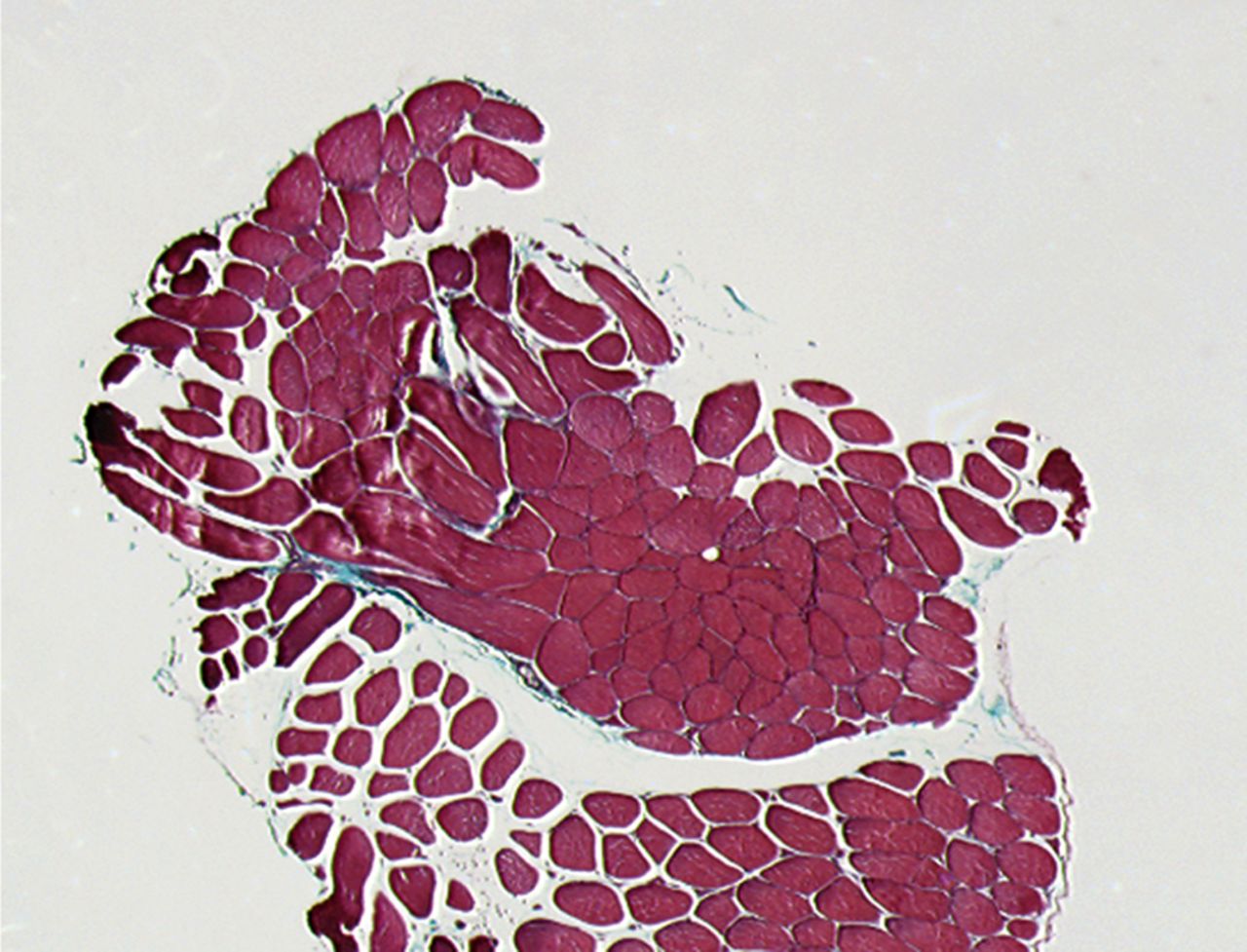
Figs. 2a - 2b
Histological images showing Masson’s trichrome staining of a representative muscle biopsy a) pre- and b) three months post-transplantation (magnification 5×).
In order to assess any regenerative potential of the injected MNCs, quantitative needle EMG and histology were performed. The mean amplitude, duration, and number of phases of the MUPs of the injured biceps muscle were compared with injured and non-injured control muscles. After three months, the increase was 36% in mean amplitude (p = 0.045), 22% in duration (p = 0.005) and 29% in number of phases (p = 0.002). The increase in number of phases were sustained at six months follow-up (p = 0.001). A dose-effect relation between group A, B and C was observed only in the mean amplitude (p = 0.03). The non-injured contralateral biceps muscle, the injured (non-injected) brachialis muscle and non-injured brachialis muscle showed no significant changes in amplitude, duration, or number of MUP phases. The mean number of analysed MUPs was 5.3 (sd 2.2). This low number represents the severity of the nerve lesions in which patients were unable to recruit more motor units (Fig. 3).
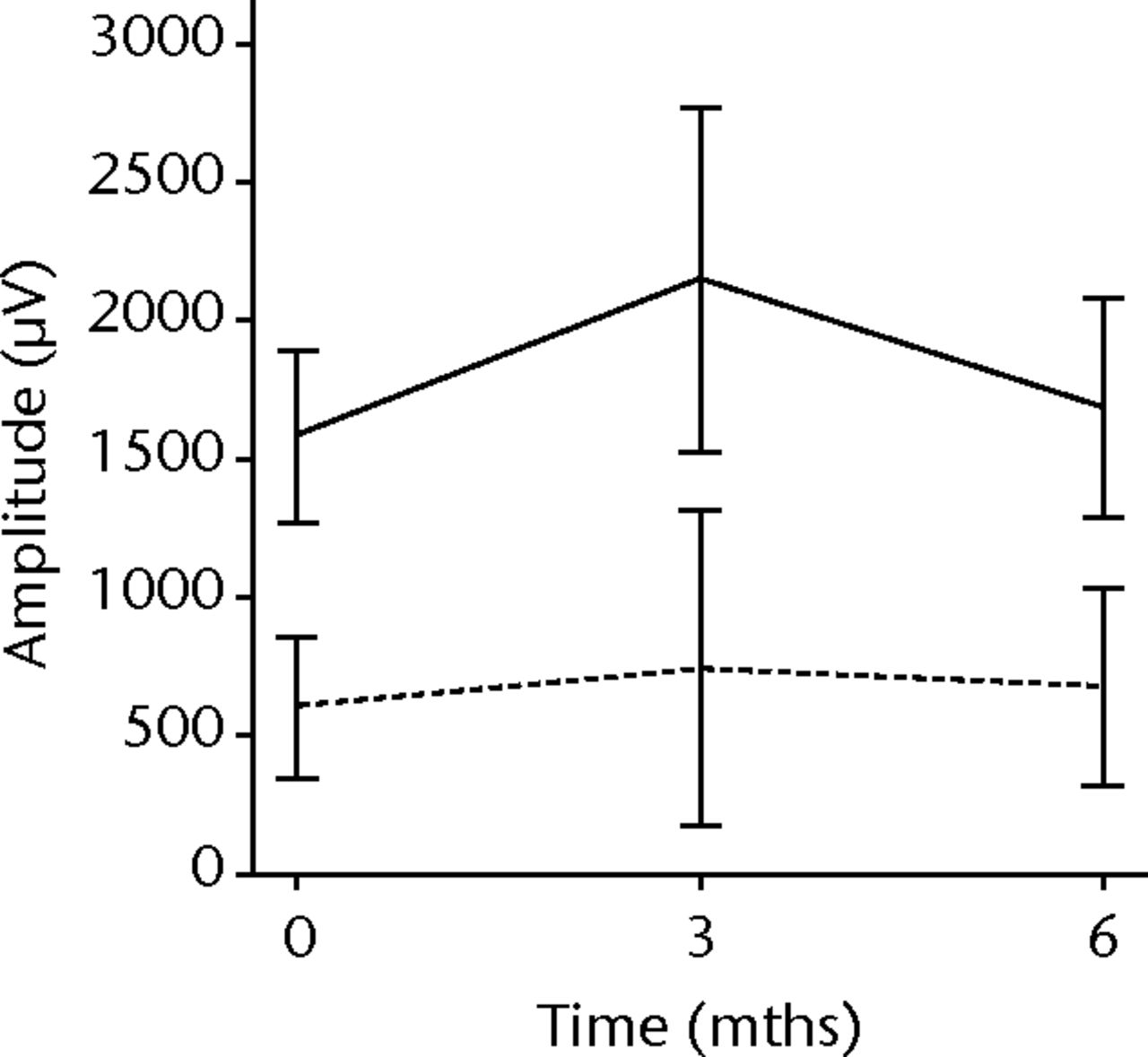
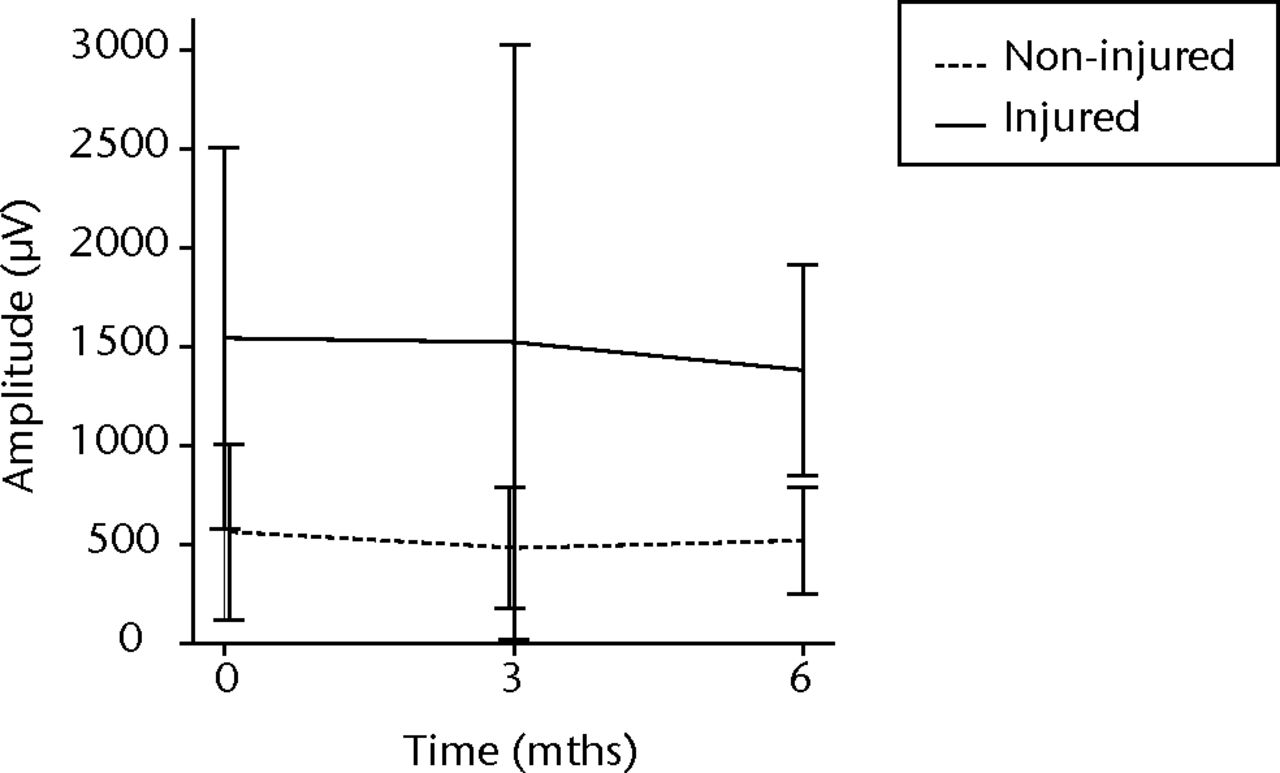
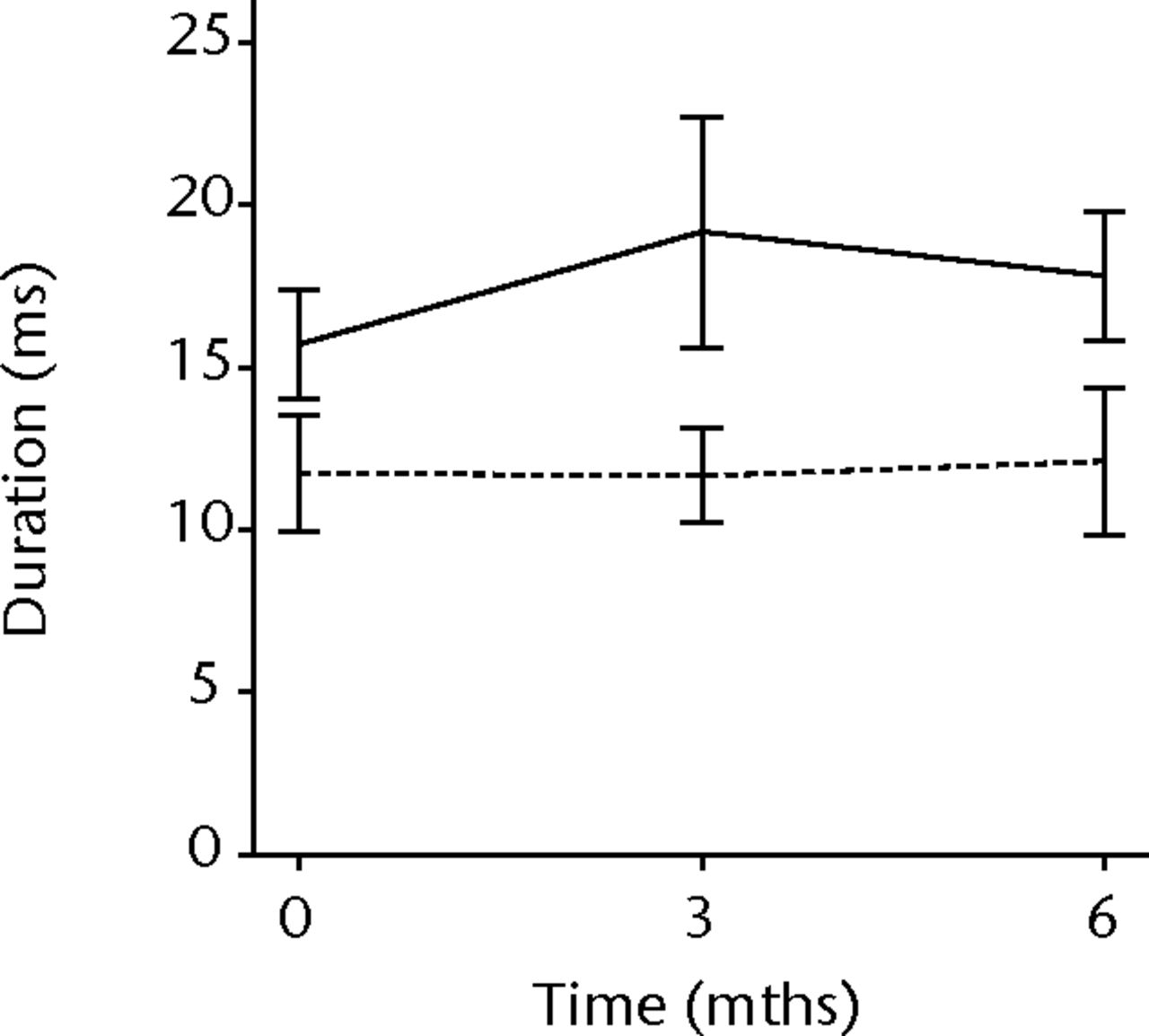
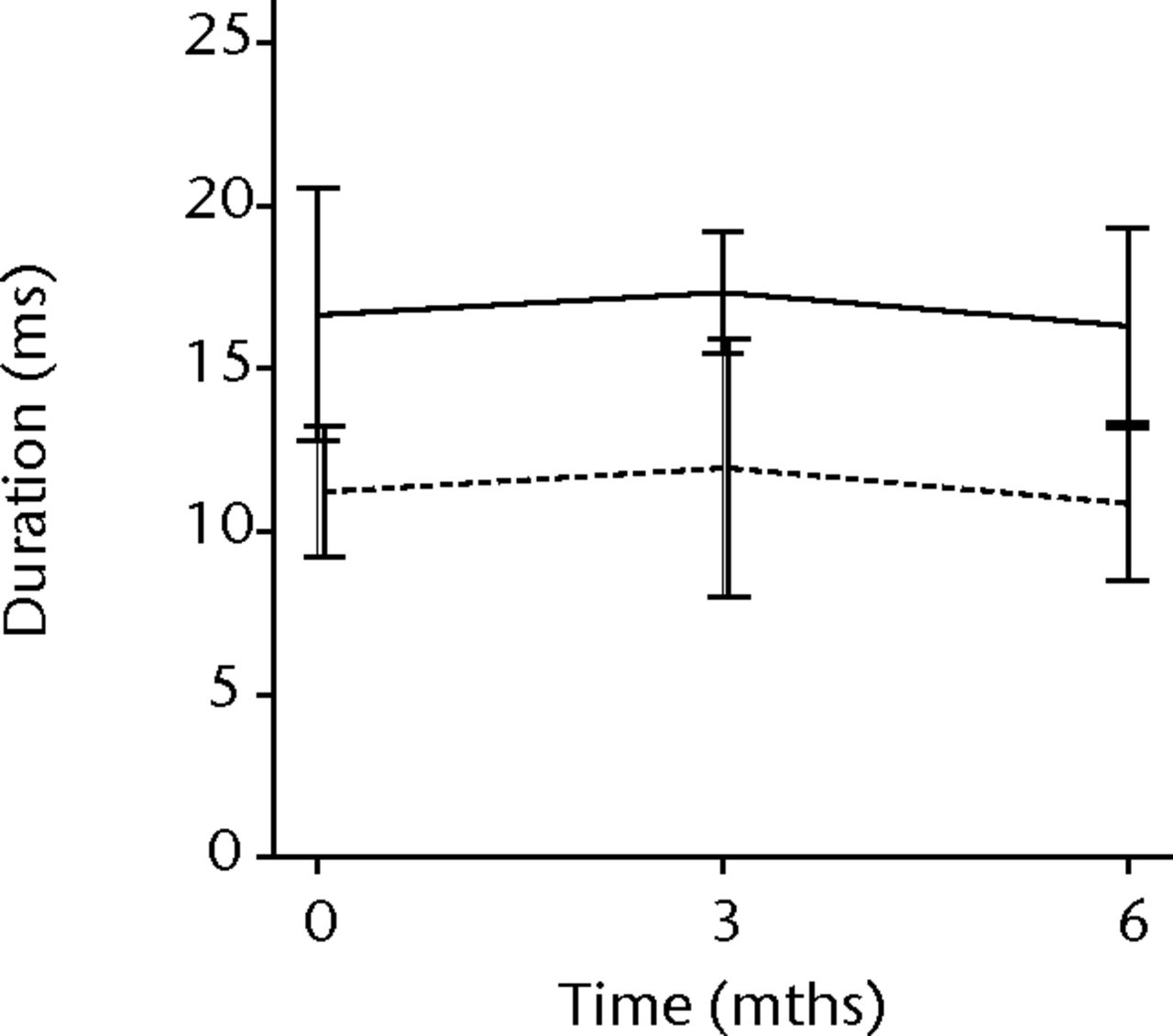
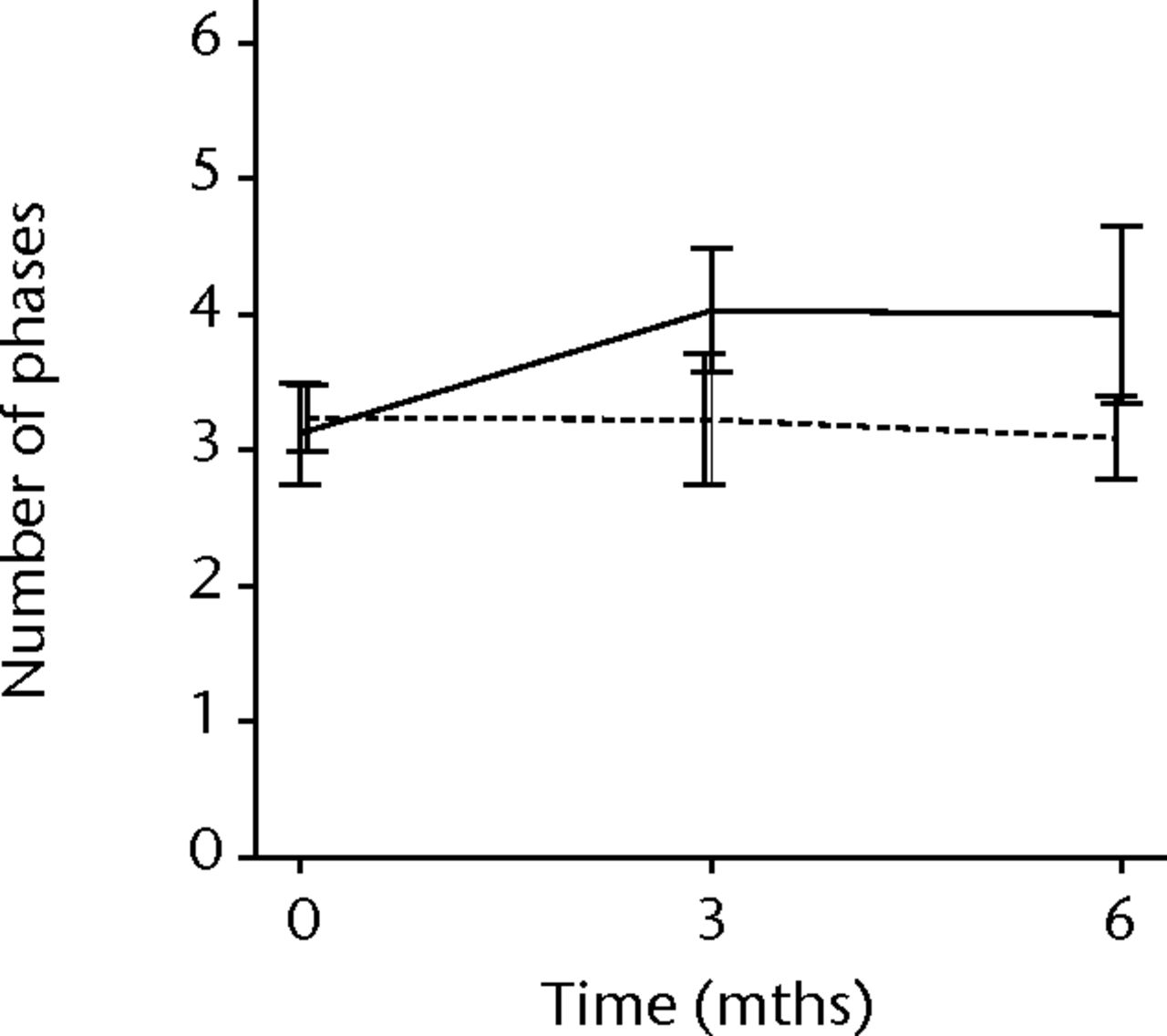
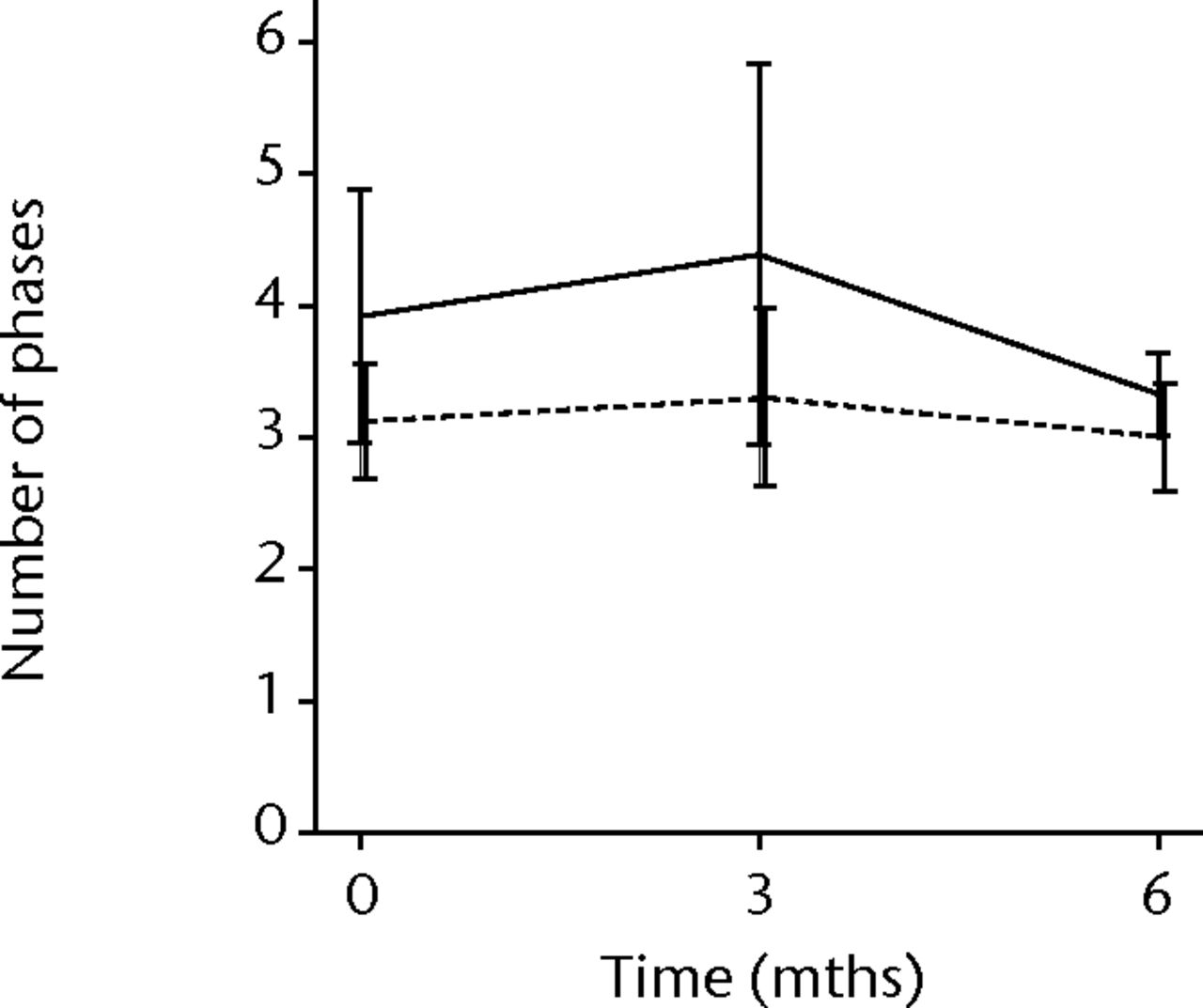
Figs. 3a - 3f
Graphs showing the mean amplitude (a and b), duration (c and d) and number of phases (e and f) of motor unit potentials of the injured and non-injured biceps (left column) and brachialis muscle (right column) pre-transplantation and at three and six months follow-up for the total patient group. Error bars denote 95% confidence interval.
The myogenic repair potential of the injected MNC cells was also evaluated using the histology of the injured biceps muscle. Analysis of all patients showed a mean increase of 80% in myofibre diameter three months after the cell injection compared with the pre-injection biopsy (p = 0.007). Pax7 staining demonstrated a 50% increase in the number of satellite cells (p = 0.045). Von Willebrand Factor staining showed an increase of 83% in the number of capillaries per myofibre (p< 0.001). No significant increase was demonstrated in the number of centronucleated myofibres or CD56 positive myofibres after cell therapy (Table II). Histological muscle regeneration was most apparent in group B, which observed a 126% increase in myofibre diameter, a 186% increase in percentage of centronucleated myofibres, a 117% increase in number of satellite cells, a 100% increase in capillaries per myofibre and a 70% decrease in the area of fibrosis. No dose-effect relation was observed in any of the histological measurements. Figure 4 shows a representative example of H& E, Pax7, and vWF and CD56 staining of a muscle biopsy before and after cell therapy in group B. After cell therapy, the muscle biopsy of one patient in group A did not contain 200 muscle fibres and was therefore excluded from analysis. In group A, no analysis of the CD56 staining could be performed because one patient’s muscle biopsy after cell therapy contained only 120 myofibres and was therefore excluded.
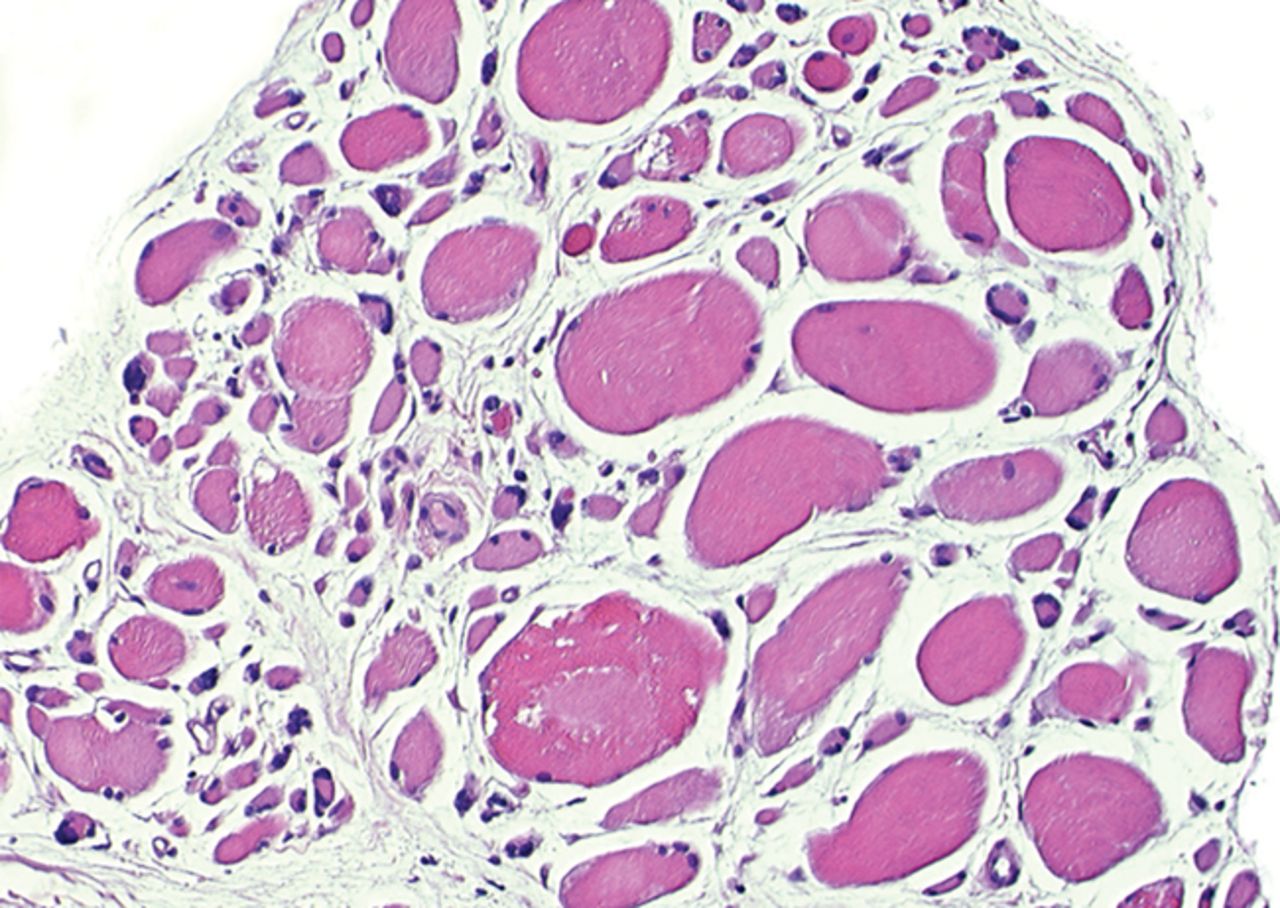
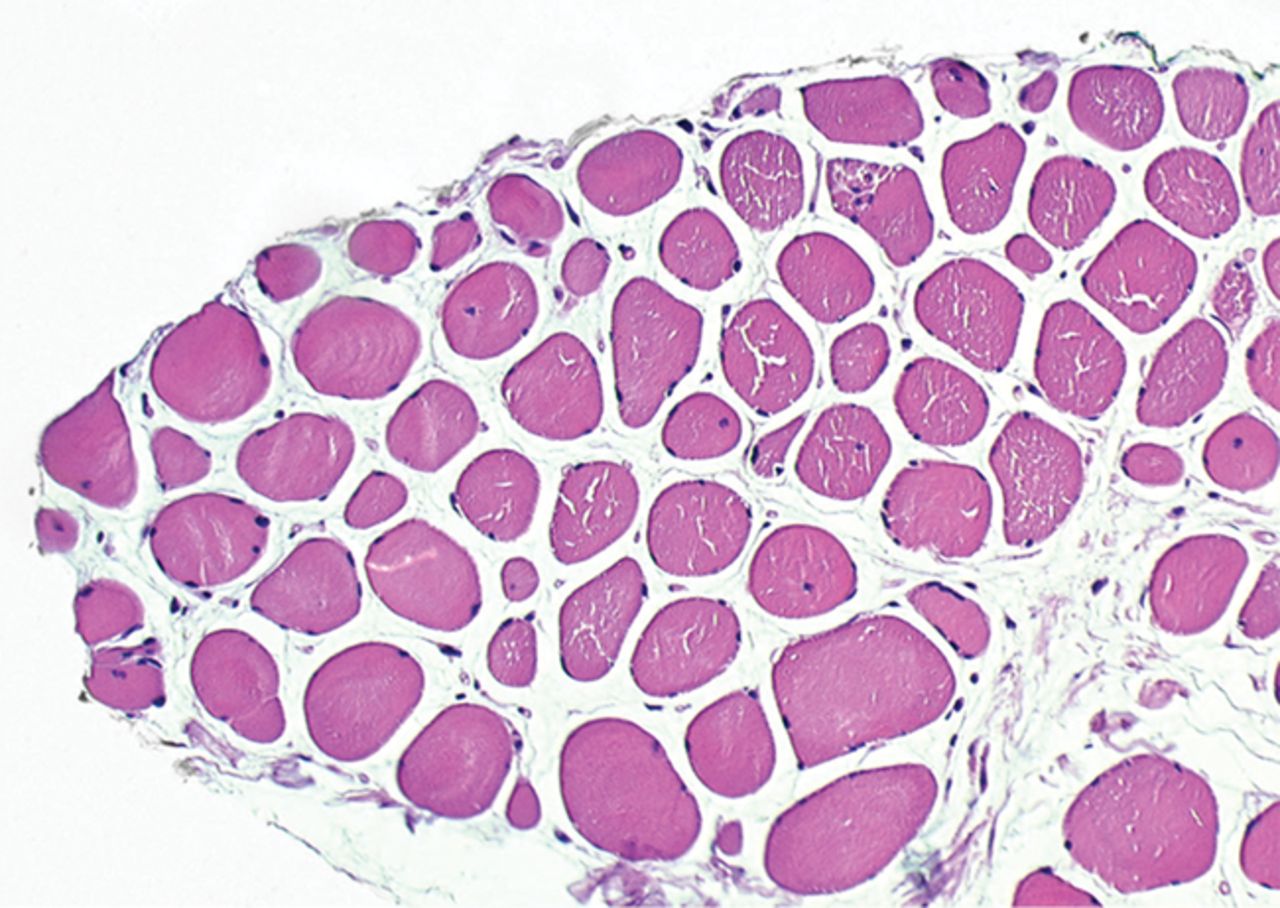
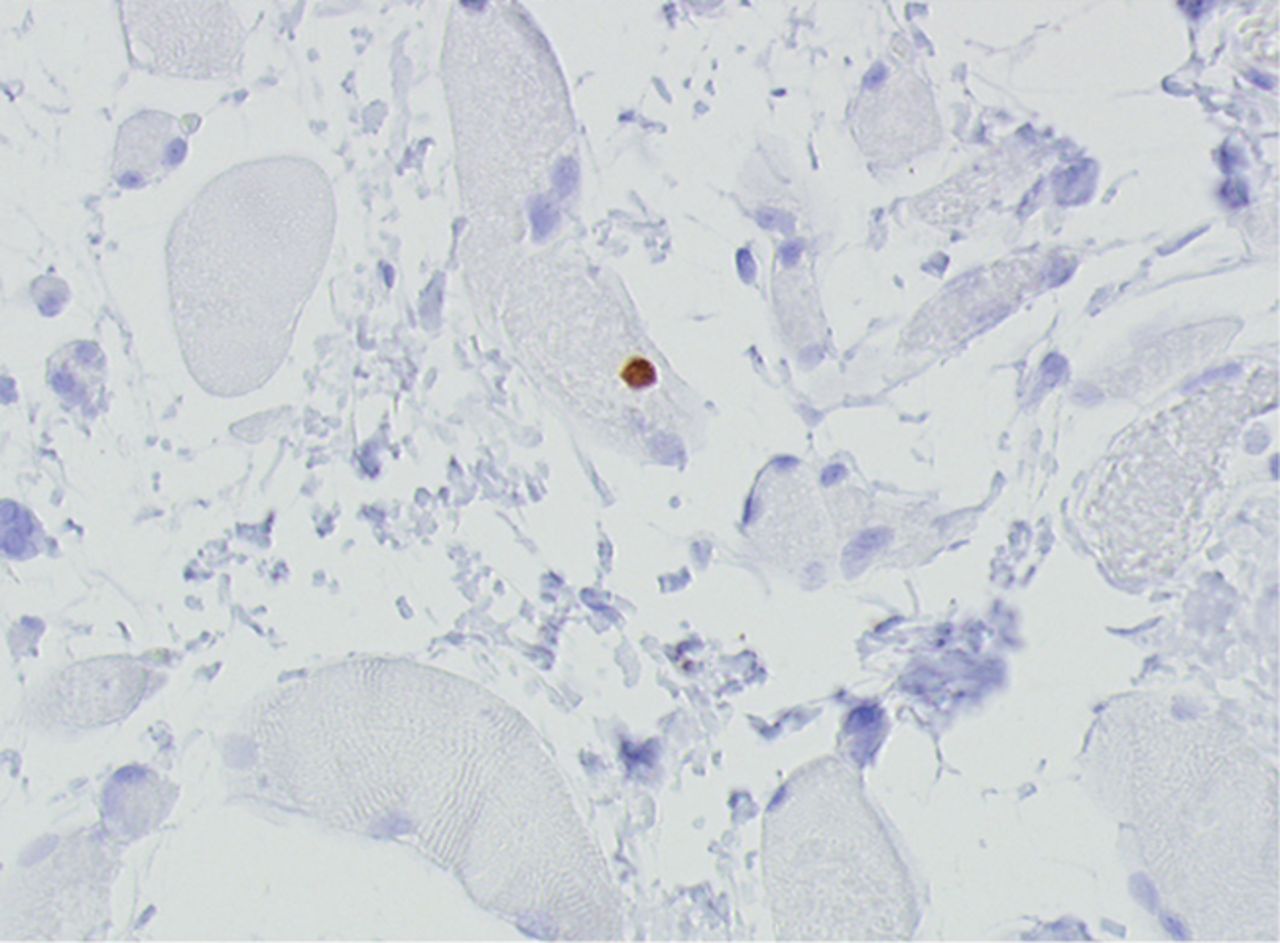
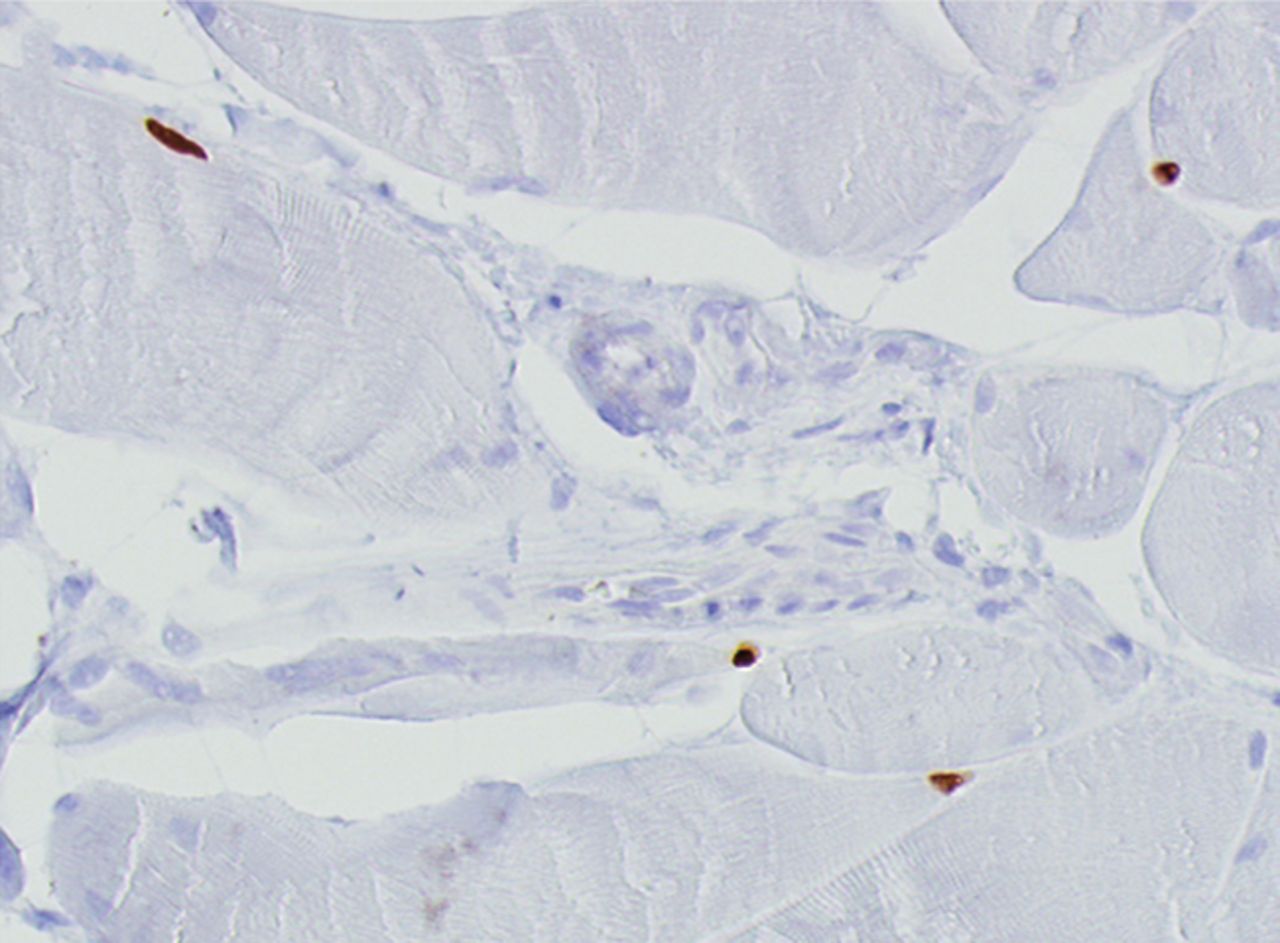
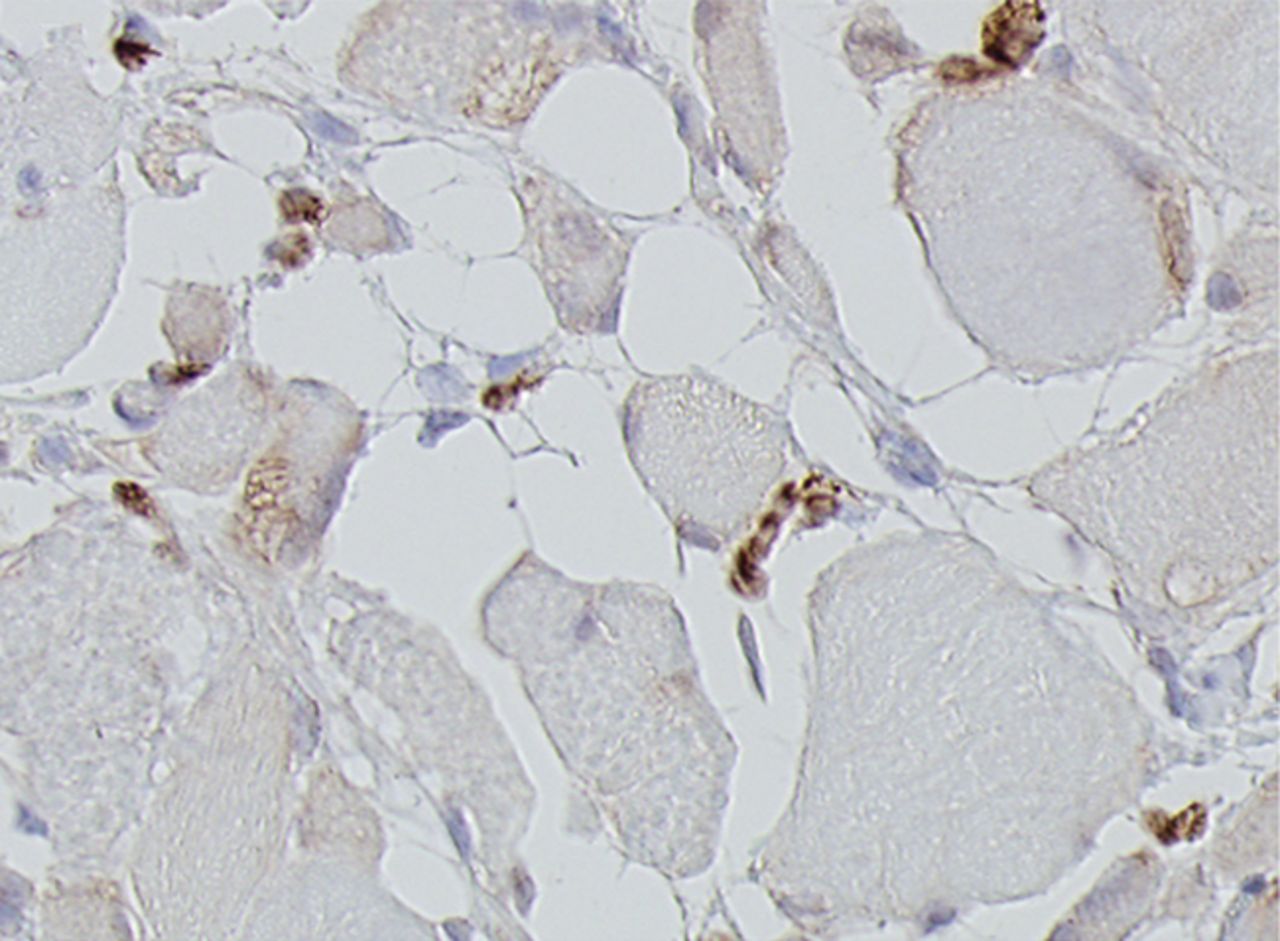
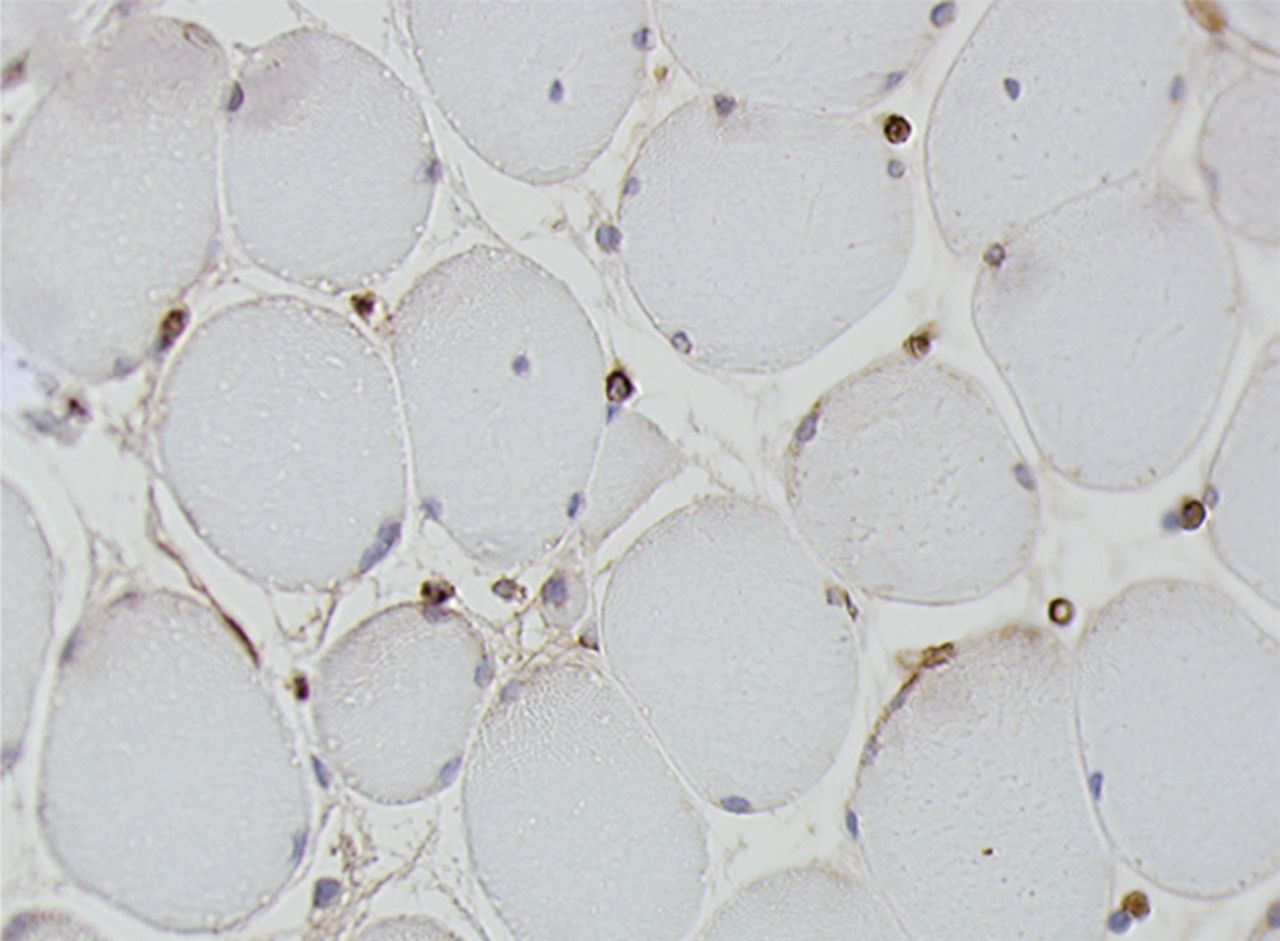
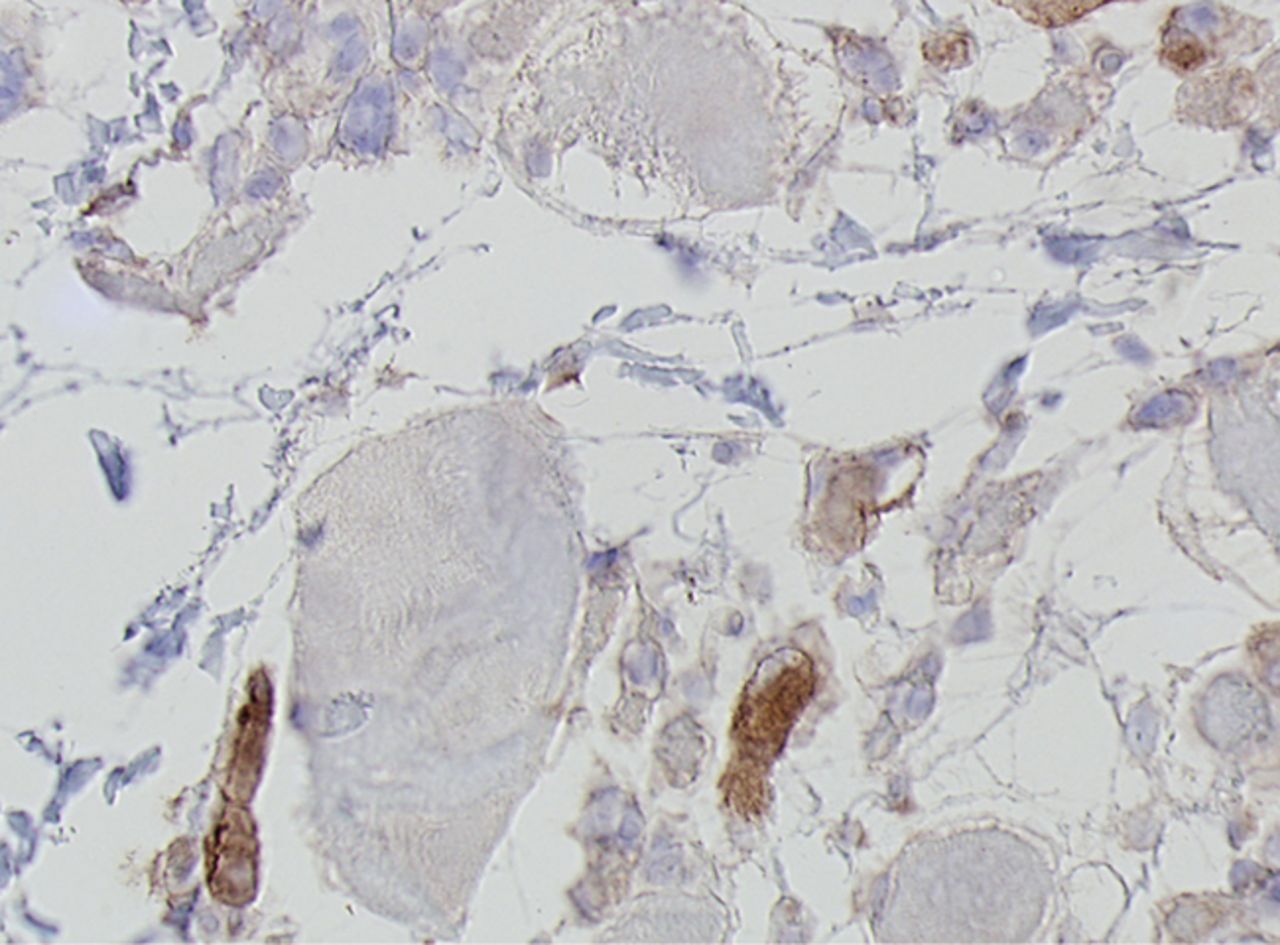
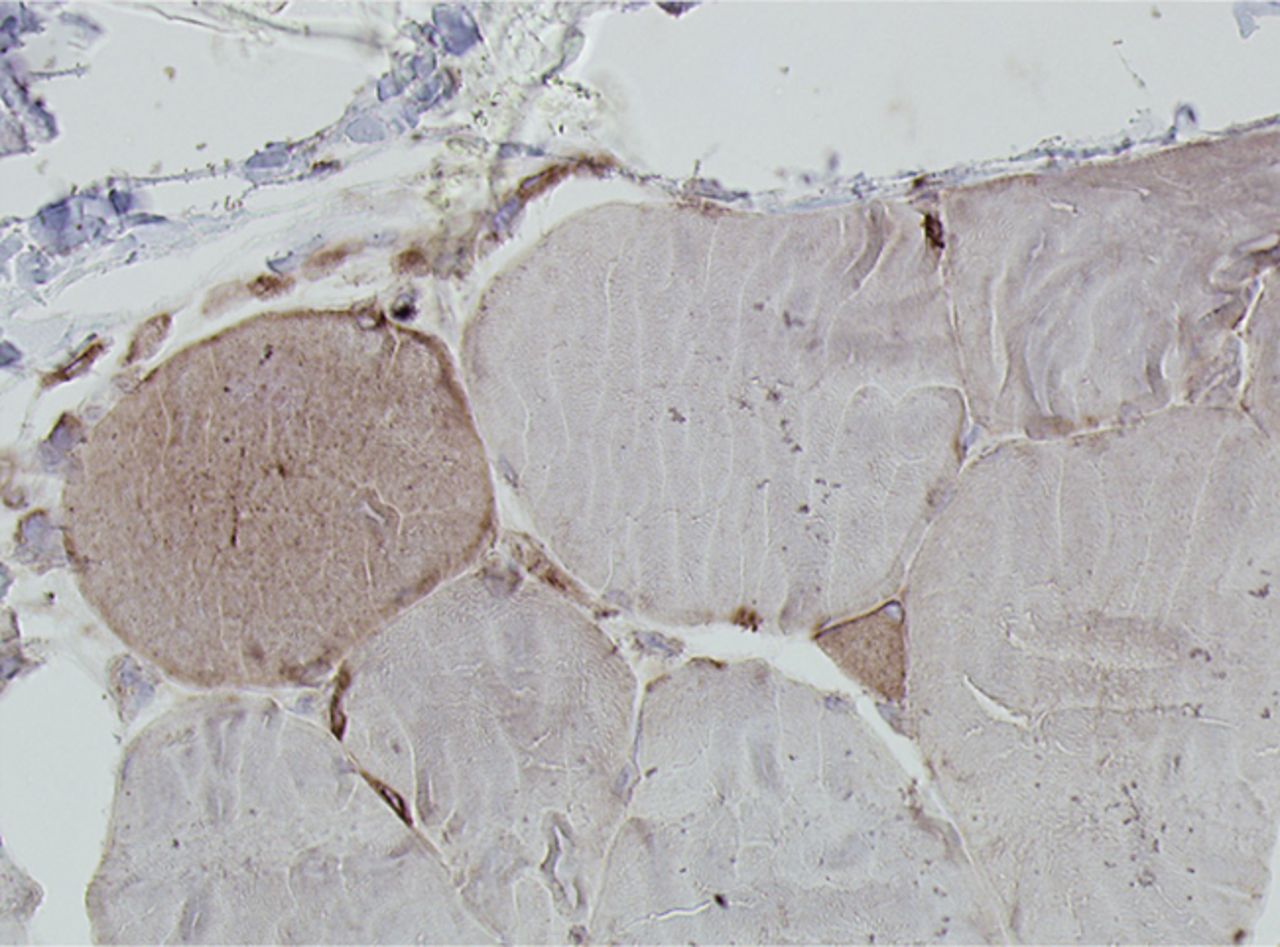
Figs. 4a - 4h
Histological images for specimens taken pre-transplantation (left column) and at three months follow-up (right column), showing staining of a representative muscle biopsy with haematoxylin and eosin (a and b; ×20), Pax7 (c and d, ×40), vWF (e and f, ×40) and CD56 (g and h, ×40).
Table II
Mean myofibre diameter, centro-nuclear myofibres, fibrosis, Pax7+ myofibres, vWF per myofibre and CD56+ myofibres of muscle biopsies of the injured biceps muscle before MNC injection and at three months follow-up
Group | |||||
---|---|---|---|---|---|
Mean (sd) biceps histology | A (n = 3) | B (n = 3) | C (n = 3) | p-value* | Total (n = 9) |
Myofibre diameter (µm) | |||||
0 months | 254 (68) | 173 (46) | 171 (12) | 193 (53) | |
3 months | 392 (165) | 391 (44) | 274 (71) | 347 (98) | |
Mean difference | +138 | +218 | +103 | 0.39 | 0.007† |
Centro-nuclear myofibres (%) | |||||
0 months | 5.1 (2.5) | 2.1 (1.5) | 2.6 (1.7) | 3.1 (2.0) | |
3 months | 2.7 (0.8) | 6.0 (3.1) | 3.4 (1.9) | 4.2 (2.5) | |
Mean difference | -2.4 | +3.9 | +0.8 | 0.01 | 0.30† |
Fibrosis (%) | |||||
0 months | 18 (2) | 31 (2) | 23 (4) | 25 (6) | |
3 months | 17 (15) | 9 (4) | 12 (2) | 12 (7) | |
Mean difference | -1 | -22 | -11 | 0.06 | 0.01† |
Pax7+ myofibres (%) | |||||
0 months | 17 (4) | 6 (3) | 14 (6) | 12 (6) | |
3 months | 18 (8) | 13 (3) | 23 (10) | 18 (8) | |
Mean difference | +1 | +7 | +9 | 0.45 | 0.045† |
vWF+ per myofibre (%) | |||||
0 months | 0.8 (0.04) | 0.5 (0.1) | 0.5 (0.1) | 0.6 (0.2) | |
3 months | 1.1 (0.2) | 1.0 (0.2) | 1.1 (0.4) | 1.1 (0.2) | |
Mean difference | +0.3 | +0.5 | +0.6 | 0.33 | < 0.001† |
CD56+ myofibres (%) | |||||
0 months | 16 (n/a)‡ | 18 (6) | 25 (3) | 21 (5)§ | |
3 months | 16 (n/a)‡ | 18 (8) | 21 (12) | 19 (7)§ | |
Mean difference | 0 | 0 | -4 | 0.94 | 0.60† |
-
* comparison of mean differences between time points between the groups (one-way analysis of variance (ANOVA) † difference between time points for all patients (paired t-test) ‡ standard deviation not available as only one observation was made § for total seven patients
In order to observe function improvement after MNC injection, we measured the active flexion ROM and strength of the injured elbow before and after cell therapy compared with the injured and non-injured control muscles. In addition, quality of life questionnaires (SF-36 and DASH) and the VAS score for pain were used. Overall, all patients had a significant increase in active flexion ROM and flexion strength. The flexion ROM increase was 152% at three months (p < 0.001) and 147% at six months follow-up (p< 0.001). The flexion strength increase was 38% at three months (p< 0.001) and 46% at six months follow-up (p< 0.001). As expected, the increase in active elbow flexion ROM and flexion strength was most prominently significant in patient groups where cell therapy was combined with a modified Steindler procedure (groups B and C). No significant changes in SF-36, DASH or VAS score were observed comparing pre- and post-injection at three months (Table III).
Table III
Elbow flexion range of movement (ROM) and strength of the injured elbow together with the Short-Form 36 and Disabilities of the Arm Shoulder and Hand (DASH; Dutch language version) questionnaires and visual analogue scale (VAS) for pain before mononuclear cell (MNC) injection and after three and six months
Group | ||||||
---|---|---|---|---|---|---|
Mean (sd) outcome* | A (n = 3) | B (n = 3) | C (n = 3) | p-value† | Total (n = 9) | |
Flexion ROM (°) | 0.22 | |||||
0 months | 65 (38) | 12 (20) | 30 (52) | 36 (41) | ||
3 months | 82 (28) | 115 (5) | 107 (15) | 101 (22) | ||
6 months | 88 (20) | 100 (20) | 110 (22) | 99 (20) | ||
p-value‡ | < 0.001‡ | |||||
Median flexion strength (MRC grade) (range) | 0.02 | |||||
0 months | 3 (3 to 3) | 2 (2 to 3) | 2 (2 to 3) | 2 (2 to 3) | ||
3 months | 3 (3 to 3) | 4 (3 to 4) | 4 (3 to 4) | 3 (3 to 4) | ||
6 months | 3 (3 to 4) | 4 (4 to 4) | 4 (4 to 4) | 4 (3 to 4) | ||
p-value‡ | < 0.001‡ | |||||
SF-36 | 0.06 | |||||
0 months | 86 (6) | 57 (16) | 69 (6) | 78 (15) | ||
3 months | 91 (5) | 71 (18) | 74 (9) | 78 (14) | ||
6 months | 82 (11) | 72 (15) | 75 (10) | 76 (12) | ||
p-value‡ | 0.06‡ | |||||
DASH-DLV | 0.15 | |||||
0 months | 43 (19) | 35 (25) | 75 (16) | 51 (25) | ||
3 months | 64 (39) | 68 (19) | 76 (12) | 69 (23) | ||
6 months | 41 (16) | 71 (17) | 74 (16) | 65 (20) | ||
p-value‡ | 0.09‡ | |||||
VAS for pain | 0.02 | |||||
0 months | 0.8 (0.8) | 6.1 (2.1) | 1.0 (1.1) | 2.7 (2.9) | ||
3 months | 0.8 (0.6) | 2.3 (2.0) | 1.3 (1.6) | 1.5 (1.5) | ||
6 months | 0.8 (0.6) | 2.0 (0.5) | 2.2 (3.0) | 1.7 (1.7) | ||
p-value‡ | 0.24‡ | |||||
-
* MRC, Medical Research Council; SF-36, Short-Form 36 (scored from 0 to 100); DASH-DLV, Disabilities of the Arm Shoulder and Hand – Dutch language version (scored from 0 to 100); VAS, visual analogue scale (scored from 0 (no pain) to 10 (extreme pain) † comparison of differences between pre-op and six months in the groups (mixed model analysis) ‡ difference between pre-op and six month values for all patients (mixed model analysis)
Discussion
Autologous BM-derived MNC injection in a partially denervated biceps muscleis safe and shows no adverse events with respect to vital signs, BM aspiration sites, injection sites or surgical wounds. Only a mild anaemia was observed, which recovered fully. The interstitial fibrosis present at long-term denervation decreased by 52% after MNC injection. These results run parallel to the results of CT scan analysis, which demonstrated a decrease of 48% in MMD.
Our secondary aim was to estimate myogenic repair and reinnervation of partial denervated muscles after MNC injection. Histological analysis, quantitative needle EMG and CT scan analysis demonstrated muscle improvement after MNC injection, which was not present in the control muscles of the sound arm or in the non-injected injured muscles of the affected arm.
Our study has some limitations. While useful to quantify fatty degeneration of rotator cuff muscles, CT analysis only provides an indirect measure of the amount of muscle lipid or fibrosis.33 We observed a significant decrease in MMD of the injured biceps muscles after cell injection, which could reflect decrease in muscle fibrosis and increase in muscle vascularisation, muscle fattening or oedema from the procedure.36 Histology of the muscle biopsies demonstrated a 52% decrease in muscle fibrosis. In addition, an 83% increase in capillaries per myofibre was observed. Using routine H& E staining, no increase in muscle fattening was seen, thus the decrease in MMD reflects either a decrease in muscle fibrosis or an increase in muscle vascularisation. The presence of muscle denervation was assessed using quantitative needle EMG. Analysing a minimum of 20 MUPs per analysed muscle is generally accepted in the literature.34,37,38 Unfortunately, we could not obtain a minimum of 20 MUPs per analysed muscle (mean 5.3 MUPs [sd 2.2]), due to the partial denervation. Nevertheless a significant difference in mean amplitude, duration and the number of phases was found.
Another limitation was that in groups B and C, the BM-derived MNC injection was combined with a flexor-pronator transfer, which improves elbow flexion. Since the flexor-pronator group has to be fixated to the distal humerus with a bicortical screw, it is possible that BM may leak underneath the screw, stimulating the muscle in its vicinity (biceps triceps). However, CT changes were not seen in the control triceps muscle, nor were EMG improvements in the injured but non-injected brachialis muscle. A final limitation was low number of patients and lack of a control patient group (i.e., modified Steindler in a partially denervated biceps muscle with no MNC injection).
Quantitative needle EMG was used to determine muscle innervation. After injection of BM-derived MNC cells, an increase of 36% in amplitude, 22% in duration, and 29% in number of phases of MUPs was observed, suggestive for muscle reinnervation. Both human and animal studies have shown that BM stromal cells can differentiate into neural cells and improve nerve regeneration.39-42
Histology was used to evaluate the myogenic repair on a microscopic level. Upon muscle denervation, animal studies have shown a decrease from 2104 µm to 50 µm in myofibre diameter, a decrease from 1.6% to 0.2% in capillary-to-myofibre ratio and an increase from 11% to 35% in fibrosis.14,17,18 Our data are consistent with these studies, demonstrating a mean myofibre diameter of 193 µm, a mean capillary-to-myofibre ratio of 0.6 and a mean fibrosis of 25% before cell injection.
The number of cells in the satellite pool within a muscle appears to be correlated to postnatal growth characteristics of the muscle (Pax7). In adult muscle, up to 10% Pax7 positive myofibres have been reported.16,17,43 Before cell injection, we observed mean Pax7 positive myofibres of 11.9% (sd 6.5). This higher percentage of Pax7 fibres is probably due to the partially denervated biceps muscle in the current study.
After MNC injection, we observed an increase of 50% in Pax7 positive myofibres and an increase of 83% in vWF positive capillaries per myofibre. This may demonstrate that MNC injection can result in restoration of the satellite cell pool and vascular bed, which can play an important role in functional recovery following long-term denervation. Indeed, the increase of 80% in myofibre diameter suggested myofibre regeneration and thus restoration of muscle atrophy. Muscle regeneration was most prominently observed in patient group B, with an increase of 117% in Pax7 positive myofibres, 100% in capillary-to-myofibre ratio, 126% in myofibre diameter, 186% in centronuclear myofibres and a decrease of 70% in fibrosis. These results are suggestive of an optimal dose in group B, although a dose-effect response was not significant.
Skeletal muscle is a regenerative tissue in which mononuclear precursor cells respond to injury by dividing and fusing with damaged fibres.15 As such, it is an attractive target for cell-based therapy. Muscle fibre regeneration levels of up to 12% have been achieved by using appropriate transplant variables, including cell dose and mode of cell delivery.24 The present study is the first in which BM transplantation in a human muscle denervation model was assessed with objective measurements (histology, CT muscle and EMG). In humans, transplantation of the MNC fraction of the adult BM enhanced cardiac- and skeletal muscle function in post-ischaemic heart failure and peripheral artery disease.25-28 These effects could be attributed to the incorporation of stem cells and building muscle or by supplying the logistics required for efficient proliferation of innate cells via paracrine effects.44,45 Heterochronic parabiosis was shown to restore the regenerative potential of satellite cells, which suggests that satellite cell activity can be modulated by their microenvironment.46 Chronic inflammation does not affect the in vitro regenerative potential of human satellite cells, which underscores the in vivo influence of the microenvironment on muscle regeneration.47 Unravelling the molecular mechanism behind the observed regeneration in BP injury patients is a challenge that still has to be met. In the future, this can be studied in newly developed animal models representative for BP injury.48,49
In conclusion, BM-derived MNC injection is safe in partially denervated muscle of traumatic BP patients. Significant muscle improvement has been observed in muscle biopsies, quantitative needle EMG and CT scan analysis. Although promising, the preliminary results of the present study require confirmation in a larger controlled clinical study.
Acknowledgements: We thank A. Kawakami for the Pax7 antibody, obtained from the Developmental Studies Hybridoma Bank, developed under the auspices of the NICHD and maintained by the University of Iowa, Department of Biological Sciences, Iowa City, Iowa. The assistance on the research protocol provided by J. H.N. Lindeman was greatly appreciated. We thank F. A. Prins, I. M. Hegeman and C. Welling of the Department of Pathology, Leiden University Medical Center, Leiden, The Netherlands for their technical assistance.
1 Midha R . Epidemiology of brachial plexus injuries in a multitrauma population. Neurosurgery1997;40:1182–1188.CrossrefPubMed Google Scholar
2 Asplund M , NilssonM, JacobssonA, von HolstH. Incidence of traumatic peripheral nerve injuries and amputations in Sweden between 1998 and 2006. Neuroepidemiology2009;32:217–228.CrossrefPubMed Google Scholar
3 Waters PM . Update on management of pediatric brachial plexus palsy. J Pediatr Orthop B2005;14:233–244.CrossrefPubMed Google Scholar
4 Kim DH , ChoYJ, TielRL, KlineDG. Outcomes of surgery in 1019 brachial plexus lesions treated at Louisiana State University Health Sciences Center. J Neurosurg2003;98:1005–1016.CrossrefPubMed Google Scholar
5 Nagano A . Treatment of brachial plexus injury. J Orthop Sci1998;3:71–80.CrossrefPubMed Google Scholar
6 Malessy MJ , de RuiterGC, de BoerKS, ThomeerRT. Evaluation of suprascapular nerve neurotization after nerve graft or transfer in the treatment of brachial plexus traction lesions. J Neurosurg2004;101:377–389.CrossrefPubMed Google Scholar
7 Krishnan KG , MartinKD, SchackertG. Traumatic lesions of the brachial plexus: an analysis of outcomes in primary brachial plexus reconstruction and secondary functional arm reanimation. Neurosurgery2008;62:873–885.CrossrefPubMed Google Scholar
8 Monreal R . Steindler flexorplasty to restore elbow flexion in C5-C6-C7 brachial plexus palsy type. J Brachial Plex Peripher Nerve Inj2007;2:15.CrossrefPubMed Google Scholar
9 Ishida O , SunagawaT, SuzukiO, OchiM. Modified Steindler procedure for the treatment of brachial plexus injuries. Arch Orthop Trauma Surg2006;126:63–65.CrossrefPubMed Google Scholar
10 Brunelli GA , VigasioA, BrunelliGR. Modified Steindler procedure for elbow flexion restoration. J Hand Surg Am1995;20:743–746.CrossrefPubMed Google Scholar
11 Lu DX , HuangSK, CarlsonBM. Electron microscopic study of long-term denervated rat skeletal muscle. Anat Rec1997;248:355–365.CrossrefPubMed Google Scholar
12 Borisov AB , DedkovEI, CarlsonBM. Differentiation of activated satellite cells in denervated muscle following single fusions in situ and in cell culture. Histochem Cell Biol2005;124:13–23.CrossrefPubMed Google Scholar
13 Borisov AB , DedkovEI, CarlsonBM. Abortive myogenesis in denervated skeletal muscle: differentiative properties of satellite cells, their migration, and block of terminal differentiation. Anat Embryol (Berl)2005;209:269–279.CrossrefPubMed Google Scholar
14 Ashley Z , SutherlandH, LanmullerH, et al.Atrophy, but not necrosis, in rabbit skeletal muscle denervated for periods up to one year. Am J Physiol Cell Physiol2007;292:C440–C451. Google Scholar
15 Hawke TJ , GarryDJ. Myogenic satellite cells: physiology to molecular biology. J Appl Physiol2001;91:534–551.CrossrefPubMed Google Scholar
16 Viguie CA , LuDX, HuangSK, RengenH, CarlsonBM. Quantitative study of the effects of long-term denervation on the extensor digitorum longus muscle of the rat. Anat Rec1997;248:346–354.CrossrefPubMed Google Scholar
17 Dedkov EI , KostrominovaTY, BorisovAB, CarlsonBM. Reparative myogenesis in long-term denervated skeletal muscles of adult rats results in a reduction of the satellite cell population. Anat Rec2001;263:139–154.CrossrefPubMed Google Scholar
18 Borisov AB , HuangSK, CarlsonBM. Remodeling of the vascular bed and progressive loss of capillaries in denervated skeletal muscle. Anat Rec2000;258:292–304.CrossrefPubMed Google Scholar
19 Lazerges C , DaussinPA, CouletB, et al.Transplantation of primary satellite cells improves properties of reinnervated skeletal muscles. Muscle Nerve2004;29:218–226.CrossrefPubMed Google Scholar
20 Skuk D . Myoblast transplantation for inherited myopathies: a clinical approach. Expert Opin Biol Ther2004;4:1871–1885.CrossrefPubMed Google Scholar
21 Beauchamp JR , MorganJE, PagelCN, PartridgeTA. Dynamics of myoblast transplantation reveal a discrete minority of precursors with stem cell-like properties as the myogenic source. J Cell Biol1999;144:1113–1122.CrossrefPubMed Google Scholar
22 Matziolis G , WinklerT, SchaserK, et al.Autologous bone marrow-derived cells enhance muscle strength following skeletal muscle crush injury in rats. Tissue Eng2006;12:361–367.CrossrefPubMed Google Scholar
23 Ferrari G , Cusella-DeAG, ColettaM, et al.Muscle regeneration by bone marrow-derived myogenic progenitors. Science1998;279:1528–1530.CrossrefPubMed Google Scholar
24 Abedi M , GreerDA, ColvinGA, et al.Robust conversion of marrow cells to skeletal muscle with formation of marrow-derived muscle cell colonies: a multifactorial process. Exp Hematol2004;32:426–434.CrossrefPubMed Google Scholar
25 Van Tongeren RB , HammingJF, FibbeWE, et al.Intramuscular or combined intramuscular/intra-arterial administration of bone marrow mononuclear cells: a clinical trial in patients with advanced limb ischemia. J Cardiovasc Surg (Torino)2008;49:51–58. Google Scholar
26 Tateishi-Yuyama E , MatsubaraH, MuroharaT, et al.Therapeutic angiogenesis for patients with limb ischaemia by autologous transplantation of bone-marrow cells: a pilot study and a randomised controlled trial. Lancet2002;360:427–435.CrossrefPubMed Google Scholar
27 Kamihata H , MatsubaraH, NishiueT, et al.Implantation of bone marrow mononuclear cells into ischemic myocardium enhances collateral perfusion and regional function via side supply of angioblasts, angiogenic ligands, and cytokines. Circulation2001;104:1046–1052.CrossrefPubMed Google Scholar
28 Beeres SL , BaxJJ, bbets-SchneiderP, et al.Intramyocardial injection of autologous bone marrow mononuclear cells in patients with chronic myocardial infarction and severe left ventricular dysfunction. Am J Cardiol2007;100:1094–1098.CrossrefPubMed Google Scholar
29 No authors listed. Aids to the examination of the peripheral nervous system (Memorandum no. 45). Fourth ed. London: W. B. Saunders, 2000. Google Scholar
30 Brunelli GA , VigasioA, BrunelliGR. Modified Steindler procedure for elbow flexion restoration. J Hand Surg Am1995;20:743–746.CrossrefPubMed Google Scholar
31 Veehof MM , SleegersEJ, van VeldhovenNH, SchuurmanAH, van MeeterenNL. Psychometric qualities of the Dutch language version of the Disabilities of the Arm, Shoulder, and Hand questionnaire (DASH-DLV). J Hand Ther2002;15:347–354.CrossrefPubMed Google Scholar
32 McHorney CA , WareJE Jr, LuJF, SherbourneCD. The MOS 36-item Short-Form Health Survey (SF-36): III. Tests of data quality, scaling assumptions, and reliability across diverse patient groups. Med Care1994;32:40–66.CrossrefPubMed Google Scholar
33 van de Sande MA , StoelBC, ObermannWR, LiengJG, RozingPM. Quantitative assessment of fatty degeneration in rotator cuff muscles determined with computed tomography. Invest Radiol2005;40:313–319.CrossrefPubMed Google Scholar
34 Stålberg E , FalckB, SonooM, StålbergS, AströmM. Multi-MUP EMG analysis: a two year experience in daily clinical work. Electroencephalogr Clin Neurophysiol1995;97:145–154. Google Scholar
35 Dorph C , NennesmoI, LundbergIE. Percutaneous conchotome muscle biopsy: a useful diagnostic and assessment tool. J Rheumatol2001;28:1591–1599. Google Scholar
36 Gargiulo P , HelgasonT, IngvarssonP, et al.Medical image analysis and 3-D modelling to quantify changes and functional restoration in denervated muscle undergoing electrical stimulation treatment. Human-centric Computing and Information Sciences2012;2:10. Google Scholar
37 Nandedkar SD , BarkhausPE, CharlesA. Multi-motor unit action potential analysis (MMA). Muscle Nerve1995;18:1155–1166.PubMed Google Scholar
38 Bischoff C , MachetanzJ, MeyerBU, ConradB. Repetitive magnetic nerve stimulation: technical considerations and clinical use in the assessment of neuromuscular transmission. Electroencephalogr Clin Neurophysiol1994;93:15–20.CrossrefPubMed Google Scholar
39 Braga-Silva J , GehlenD, PadoinAV, et al.Can local supply of bone marrow mononuclear cells improve the outcome from late tubular repair of human median and ulnar nerves?J Hand Surg Eur Vol2008;33:488–493.CrossrefPubMed Google Scholar
40 Cuevas P , CarcellerF, Garcia-GomezI, YanM, DujovnyM. Bone marrow stromal cell implantation for peripheral nerve repair. Neurol Res2004;26:230–232.CrossrefPubMed Google Scholar
41 Goel RK , SuriV, SuriA, et al.Effect of bone marrow-derived mononuclear cells on nerve regeneration in the transection model of the rat sciatic nerve. J Clin Neurosci2009;16:1211–1217.CrossrefPubMed Google Scholar
42 Kumar AA , KumarSR, NarayananR, ArulK, BaskaranM. Autologous bone marrow derived mononuclear cell therapy for spinal cord injury: a phase I/II clinical safety and primary efficacy data. Exp Clin Transplant2009;7:241–248.PubMed Google Scholar
43 Schultz E , ChamberlainC, McCormickKM, MozdziakPE. Satellite cells express distinct patterns of myogenic proteins in immature skeletal muscle. Dev Dyn2006;235:3230–3239.CrossrefPubMed Google Scholar
44 Gnecchi M , HeH, LiangOD, et al.Paracrine action accounts for marked protection of ischemic heart by Akt-modified mesenchymal stem cells. Nat Med2005;11:367–368.CrossrefPubMed Google Scholar
45 Heil M , ZiegelhoefferT, MeesB, SchaperW. A different outlook on the role of bone marrow stem cells in vascular growth: bone marrow delivers software not hardware. Circ Res2004;94:573–574. Google Scholar
46 Conboy IM , ConboyMJ, WagersAJ, et al.Rejuvenation of aged progenitor cells by exposure to a young systemic environment. Nature2005;433:760–764.CrossrefPubMed Google Scholar
47 Duijnisveld BJ , BigotA, BeenakkerKG, et al.Regenerative potential of human muscle stem cells in chronic inflammation. Arthritis Res Ther2011;13:R207.CrossrefPubMed Google Scholar
48 Kim HM , GalatzLM, DasR, PatelN, ThomopoulosS. Musculoskeletal deformities secondary to neurotomy of the superior trunk of the brachial plexus in neonatal mice. J Orthop Res2010;28:1391–1398.CrossrefPubMed Google Scholar
49 Li Z , MaJ, ApelP, et al.Brachial plexus birth palsy-associated shoulder deformity: a rat model study. J Hand Surg Am2008;33:308–312.CrossrefPubMed Google Scholar
Funding statement:
Dutch Arthritis Association (project number LLR13) and Translational Research of ZonMw, The Netherlands organisation for health research and development (project number 95100105)
Author contributions:
S. Hogendoorn: Design of study, Data collection, Data analysis, Writing the paper
B. J. Duijnisveld: Data collection, Data analysis, Writing the paper
S. G. van Duinen: Data collection (histology), Data analysis, Writing a part of the paper
B. C. Stoel: Data collection (quantitative CT), Data analysis, Writing a part of the paper
J. G. van Dijk: Performing the EMGs, Data collection (EMG), Data analysis, Writing a part of the paper
W. E. Fibbe: Facilitating with the stem cell laboratory (BM-derived MNC separation), Design of the study, Writing a part of the paper
R. G. H. H. Nelissen: Design of the study, Performing the surgeries, Data collection (clinical studies), Data analysis, Writing the paper
ICMJE Conflict of Interest:
None declared
©2014 The British Editorial Society of Bone & Joint Surgery. This is an open-access article distributed under the terms of the Creative Commons Attributions licence, which permits unrestricted use, distribution, and reproduction in any medium, but not for commercial gain, provided the original author and source are credited.
Supplementary material. An appendix giving further details of the methods section is available alongside this article on our website www.bjr.boneandjoint.org.uk